Within virology research, the advances in light microscopy methods and labeling techniques have permitted much better flexibility in terms of experimental capabilities. Super-resolution methods like PALM, SIM, STORM, TIRF, and SRRF-Stream+ enable the diffraction limit of light to be overcome and enhance imaging resolution down to 50 nm, or more.
Better resolution enables more insight into the dynamic and intricate nature of how viruses interact with the host cell throughout the infection cycle.
Therefore, by utilizing light microscopy methods, the range of what can be studied can span from studying the detection of virus within a sample, general histopathological effects within tissue samples, localization of viruses within cells, and potentially, tracking of live viruses during the virus infection cycle.
Sample preparation and handling
Safety is of course, is a key aspect of handling any potential samples containing a known virus within the laboratory, or indeed an unknown virus or pathogen in a clinical setting.
Samples must be prepared for analysis according to the biosafety guidelines for the virus under study. It should be noted that since a specific virus may be considered endemic within a population or present a higher risk in another, this can vary from one region to another.
The greater the biosafety level of the laboratory, the higher the restrictions there is to the equipment and, indeed, what experiments can be carried out. Usually, this will mean that before it can be used in the laboratory in labeling and imaging experiments, the virus must be inactivated (e.g., using chemical, heat, or pH treatments).
Alternatively, it may be possible to utilize a closely related “low-risk” virus, use virus-like particles (VLPs), or other strategies as a research model.
Part 1: Labeling methods
ELISA and PCR techniques can be utilized to detect viruses in a sample and make the basis of many diagnostic detection and fast screening methods for influenza, HIV, or SARS -CoV2. Yet, these methods do not let the user visualize viruses or their interactions with host cells.
So, it is not possible to understand much about the infection process itself. For this, Immunostaining or Immunofluorescence labeling can be employed so that proteins of interest, of both the cell and virus, can be labeled with antibodies which are fluorescently tagged.
To probe, and tag short sequences of the viral genetic material, single-molecule FISH (Fluorescence in situ Hybridization) techniques may also be utilized.
Firstly, the sample must be fixed to maintain the structure of the cell or tissue being examined. This involves agents like methanol, acetone, formaldehyde, or paraformaldehyde to fix the cells and virus.
Then a suitable antibody with a fluorescent label added, with the unbound antibody removed through a series of washes. The cells can then be studied by utilizing a suitable light microscopy method.
Direct immunolabeling involves the direct binding of a fluorescent label to a viral antigen. Indirect immunolabeling utilizes a secondary antibody which will bind to the virus-specific primary antibody.
This can generate a much higher signal level than direct labeling, as many secondary antibodies with the fluorescent label can potentially bind to the primary virus-specific antibody.
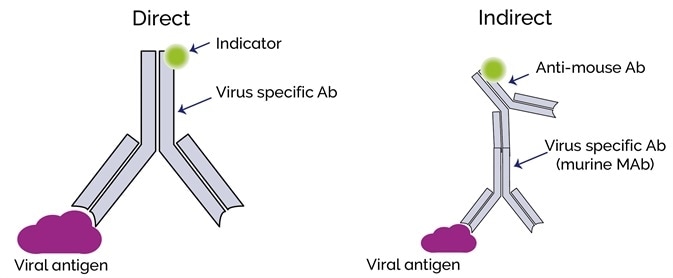
Image Credit: Andor Technology Ltd.
Live-cell imaging is more difficult than the imaging of fixed cells; with fixed cells, you may be able to utilize longer exposures and capture a stronger signal.
Yet, it is still possible to do live-imaging of viruses, and it is now possible to choose from a variety of genetically encoded fluorescent proteins to label specific viral components like envelope proteins or nucleocapsid.
Generally, immunolabeling is unsuitable for live-cell imaging, but any labeling can interfere with the viability of the virus and the normal function of the virus components. This has to be considered and validated in the experimental design.
By inserting the code for the label into the open reading frame (ORF) of the target protein, for example, a virus nucleocapsid protein, a fluorescent protein may be fused to a target protein. Ideally, this should be so there are enough copies per virion so that a useful signal level can be detected using high sensitivity EMCCD or sCMOS cameras. The host cell can also be labeled, and so, virus-host-cell interactions can be studied e.g. cell membrane, nucleus, or cytoplasm.
Varieties of labels used
Viruses are extremely small relative to the size of the cells they infect. Medium-sized viruses like Coronavirus, Adenovirus, or Influenza virus are in the order of 90-125 nm in size. Small viruses such as Parvovirus can be ~20 nm, and others as small 5 nm in size.
This means that before self-quenching effects happen to the closely bound labels, only a small number of labels can bind to the virus. As previously outlined, incorporation and expression of fluorescent proteins may also affect the normal function of the virus because of steric hindrance and the interactions with the cell.
Commonly used Fluorescent labels include:
- Fluorescent dyes which are small, organic natural, or synthetic fluorescent molecules e.g. Texas red, TRITC, fluorescein, DAPI, Cy5, and Alexa fluor
- Fluorescent Proteins are larger proteins that fluoresce because of their structure. e.g. GFP (Green Fluorescent protein).
In considering labels, options that emit more towards the red spectrum would mean that autofluorescence from the host-cell making it easier to achieve a better signal to noise from the low virus label signals against the background.
So, Cy5, mCherry, or some of the Alexa Fluor labels with longer emission wavelengths could make good candidates for labeling viruses. It should be considered that the optics of older microscopes may not be suitable for wavelengths in the Near-Infrared spectrum.
Quantum Dots (Qdots) are semiconductor crystals that are 2-10 nm in size. Qdots supply a brighter signal than conventional fluorescent labels, are stable, and have discrete emission spectra.
Due to their optical attributes, they have been widely utilized for single-molecule studies and have proven very useful. This also makes them extremely interesting for potential applications in virology studies.
Despite this, to date, there has been a slow uptake of Qdots for virology studies, partly due to challenges with labeling the viruses effectively and without perturbation of normal infectivity. Yet, this is changing and Qdots are beginning to be utilized by a growing number of research groups.
Part 2: Which imaging method is the most suitable?
The table below lists some of the main light microscopy methods compared with important experimental parameters. This can be considered as a general guide to the characteristics of different methods.
Different light microscopy techniques used across 100 recent virology publications with the iXon EMCCD camera (2017-2020) alongside the Dragonfly System and SRRF-Stream+. The applicability of each technique is compared to different experimental parameters. ■ suitable, □ some variants may be suitable.
Parameter |
Widefield Epi-fluorescence |
Confocal Laser Scanning Microscopy |
Confocal Spinning Disk Microscopy* |
Dragonfly Multimodal System |
TIRF |
Multi-photon Microscopy |
Light-Sheet (SPIM) |
SIM |
STED |
PALM/STORM |
SRRF-Stream+ |
Live Imaging Experiments |
■ |
■ |
■ |
■ |
■ |
|
■ |
□ |
■ |
■ |
■ |
Imaging over long durations |
■ |
□ |
■ |
■ |
|
|
■ |
|
|
|
■ |
High-Speed Imaging |
■ |
□ |
■ |
■ |
|
|
|
|
|
|
|
Super-resolution |
|
|
|
■ |
■ |
■ |
|
■ |
■ |
■ |
■ |
Suitable for FRET Studies |
|
■ |
■ |
■ |
■ |
■ |
|
|
|
|
|
Image in 3D |
|
□ |
■ |
■ |
■ |
■ |
■ |
■ |
|
□ |
|
Image deep Tissues |
|
|
|
■ |
|
■ |
■ |
|
|
|
|
Comments |
Limited resolution. High speeds and simple. |
Improved SNR over widefield for greater image clarity |
Dragonfly imaging system combines multiple techniques |
Improved resolution in Z-axis and can be combined with other techniques |
Useful for relatively thick samples |
Useful for relatively thick samples. Limited resolution. |
Moderate resolution improvement, using normal fluorophores |
High resolution over small field of view. Complex optical set-up. |
High resolution – but requires specific labels |
Live cell imaging at low illumination intensities with any fluorophores |
In some instances, variations of the originally listed methods may have been developed to enhance aspects like the ability to image in 3D, speed of imaging, or live cell applications.
Widefield fluorescence or more accurately epifluorescence microscopy is the most accessible and simple method as it may be carried out by utilizing a standard microscope and sensitive camera, enabling live-cell imaging at high speeds and over a depth of field and wide field of view.
Yet, it is limited to classical resolution limits, meaning that it will not be suitable when higher resolution is needed. Furthermore, the out of focus background fluorescence will decrease the signal to noise and so it will only be possible to detect very strong label signals.
By eliminating out of focus light and potentially enabling live and 3D imaging, Confocal Microscopy will further enhance the signal to noise for much better image clarity.
Confocal will also need a high sensitivity detector for the low signal levels inherent to this method and of the viruses themselves. There are two main types of confocal Microscopy – laser scanning and spinning disk.
As it enables enhanced resolution with a high signal to noise, TIRF (Total Internal Reflectance) microscopy is a common method that is utilized in virology research. The downside of this method is that the depth of the sample is limited to ~100 nm depth of the evanescent wave.
This means that TIRF is usually employed for imaging the stages of the virus cycle close to the cell membrane i.e., attachment, membrane fusion, and egress of viral progeny from the host cell.
Other super-resolution techniques - STORM, STED, SIM, PALM, and SRRF-Stream+
Stochastic Optical Reconstruction Microscopy (STORM), photoactivated localization microscopy (PALM), and Stimulated emission depletion (STED) and they provide exceptional resolution abilities.
This makes it viable to image labeled viruses in a way that was previously impossible. Variants of PALM, STORM, and STED have continued to be developed so that they can enable live and/or 3D imaging. Understandably, there are sacrifices that must be made to achieve these resolutions.
PALM and STORM utilize blinking fluorophores to localize the point-source emissions from the quickly switching fluorophores from their dark state with high precision enabling a high-resolution image to be reconstructed from 100 s to 1000 s of frames.
Resolutions of 20-50 nm are possible with these methods. Since it restricts what labels can be used, the utilization of specific photo-switchable fluorophores is a limitation to these methods.
In STED, a high-intensity laser excites the sample and stimulates fluorescent emission. A second laser acts in a “donut” shape to deplete fluorescence except for that happening in a small central sub-region of ~30-80 nm. The instrument scans across the sample to create a super-resolved image.
A downside of this method is the complex optical set-up, and that high laser powers are needed, potentially causing phototoxicity to the cells. Yet, STED can produce relatively quick, high-resolution images across a small field of view suitable for some virology experiments.
SIM – Structured Illumination Microscopy is another imaging method that may be utilized for imaging host-cells and viruses. This technique utilizes an optical means to achieve enhanced resolution, unlike some other super-resolution techniques such as STORM or PALM.
In order to create optical interference, illumination is applied in a sequence of rotating spatially spaced patterns, and the Moire patterns which are produced can be processed (via Fourier-based analysis) to create an image with improved resolution.
Further variations add a further dimension, enabling 3D-SIM. The resolution of SIM of 120-150 nm is not as good as PALM, STORM, or STED. Yet, as SIM is a purely optical method, the user is not restricted to specific labels and the method can prove to be a flexible way to achieve double the resolution of epi-fluorescence microscopy.
SRRF (Super-Resolution Radial Fluctuations) is a method that utilizes the natural fluctuations in fluorescence over time to position the emission according to their radiality. SRRF-Stream+ is a further development of this method, which enables the process to be run in real-time.
100 short exposure frames will create a super-resolved image. This method can work on multiple imaging modalities – confocal, widefield, or TIRF, and depending on the sample, settings, and microscope, can enhance resolution to between 50 to 150 nm.
It enables the live imaging of cells via low illumination intensities and is highly flexible making this method potentially suitable to a number of experiments utilizing fixed or live cells.
Dragonfly – one system, multiple imaging modalities
As it permits multiple imaging modalities to be chosen depending on the specific experiment, the Andor Dragonfly system is a unique imaging solution. This means it is possible to switch between fast and convenient wide-field imaging, or increased resolution using confocal, TIRF, dSTORM or SRRF-Stream+.
The utilization of FRET for studying virus-host cell interactions
FRET (Fluorescence Resonance Energy Transfer) allows the proximity of two fluorophores to be established within nanometer precision. FRET can be utilized with confocal and TIRF imaging modalities so that it is possible to get information on spatial and temporal relationships in living cells.
Therefore, FRET is extremely useful for examining and tracking many of the dynamic interactions of virions throughout the infection cycle. There are a variety of examples of use including showing viral RNA and proteins to be co-localized with components of the host cell membrane, nucleus, or cytoskeleton, study of replication events, uncoating of capsids, and assembly of virions.
Imaging detectors
The imaging detectors are a key part of microscopy methods. Evermore sensitive detector technologies have helped make these, and other light microscopy methods, applicable to virology studies which usually have very weak signal levels.
EMCCD detectors like the Andor iXon cameras have been widely utilized. These cameras can detect single photons and so are ideal for the demands of confocal, TIRF, and STORM.
In recent years sCMOS camera technology, which is the most common detector technology for general cell biology studies, has improved further and is more relevant to a larger range of virology imaging experiments. This has been further aided by improved labeling strategies and new, brighter fluorophores.
The latest generation of back-illuminated sCMOS cameras, for example, the Sona series, provide an alternative choice to EMCCD cameras, supplying higher temporal resolution over wider fields of view.
EMCCD cameras like the latest iXon Ultra and Life EMCCD cameras remain the most suitable detector option where the weakest signals are involved, as the sensitivity remains unmatched via other competing technologies.
References and Further Reading
- Lakdawala SS, Wu Y, Wawrzusin P, Kabat J, Broadbent AJ, Lamirande EW, et al. (2014) Influenza A Virus Assembly Intermediates Fuse in the Cytoplasm. PLoS Pathog 10(3): e1003971. https://doi.org/10.1371/journal.ppat.1003971
- Brandenburg, Boerries, and Xiaowei Zhuang. “Virus trafficking - learning from single-virus tracking.” Nature reviews. Microbiology vol. 5,3 (2007): 197-208. doi:10.1038/nrmicro1615
- Yamauchi Y. Quantum dots crack the influenza uncoating puzzle. PNAS 116 (7) 2404-2406 (2019). https://doi.org/10.1073/pnas.1822089116
- Hong Z-Y, Zhang Z-L, Tang B, Ao J, Wang C, Yu C, and Pang D W. (2018) Equipping Inner Central Components of Influenza A Virus with Quantum Dots. Analytical Chemistry 90(23),14020-14028. DOI: 10.1021/acs.analchem.8b03995
- Comparison of super-resolution techniques
- Kalina L Tosheva et al 2020 Between life and death: strategies to reduce phototoxicity in super-resolution microscopy. J. Phys. D: Appl. Phys. 53 163001 https://doi.org/10.1088/1361-6463/ab6b95
- Eric Betzig, George H. Patterson, Rachid Sougrat, O. Wolf Lindwasser, Scott Olenych, Juan S. Bonifacino, Michael W. Davidson, Jennifer Lippincott-Schwartz, Harald F. Hess: Imaging Intracellular Fluorescent Proteins at Nanometer Resolution ,Science,Vol. 313 no. 5793, 2006, pp. 1642–1645. doi:10.1126/science.1127344
- Stefan W. Hell and Jan Wichmann, "Breaking the diffraction resolution limit by stimulated emission: stimulated-emission-depletion fluorescence microscopy," Opt. Lett. 19, 780-782 (1994)
- Gustafsson N. Fast live-cell conventional fluorophore nanoscopy with ImageJ through super-resolution radial fluctuations. Nat. Commun. 2016;7(12471):12471.
- Maolin Lu, Xiaochu Ma, Walther Mothes, Chapter Eight - Illuminating the virus life cycle with single-molecule FRET imaging, Editor(s): Félix A. Rey, Advances in Virus Research, Academic Press, Volume 105, 2019, Pages 239-273, ISSN 0065-3527, ISBN 9780128184561.
Acknowledgments
Produced from materials originally authored by Dr. Alan Mullan from Oxford Instruments Andor.
About Andor Technology
Andor Technology, an Oxford Instruments company, is a global leader in the pioneering and manufacturing of high-performance scientific imaging cameras, spectroscopy solutions, and microscopy systems for research and OEM markets. Andor has been innovating the photonics industry for over 20 years and continues to set the standard for high-performance light measuring solutions, enabling its customers to break new ground by performing light measurements previously considered impossible. Andor’s digital cameras are allowing scientists around the world to measure light down to a single photon and capture events occurring within 1 billionth of a second.
Andor now has over 400 staff across 16 offices worldwide, distributing products to over 10,000 customers in 55 countries. Andor’s products are used in a wide range of applications including medical research to further the understanding of heart disease, cancer, and neuronal diseases such as Alzheimer’s and Parkinson’s disease. Andor also has applications for forensic science and astronomy. Through continuous dialogue with customers and strong teamwork, Andor continues to innovate ground-breaking products that improve the world in which we live.
Sponsored Content Policy: News-Medical.net publishes articles and related content that may be derived from sources where we have existing commercial relationships, provided such content adds value to the core editorial ethos of News-Medical.Net which is to educate and inform site visitors interested in medical research, science, medical devices, and treatments.