Genome editing refers to the engineering of intracellular DNA by modifying specific sequences of data, such as inserting, deleting, integrating or substituting nucleotides or sequences of nucleotides.
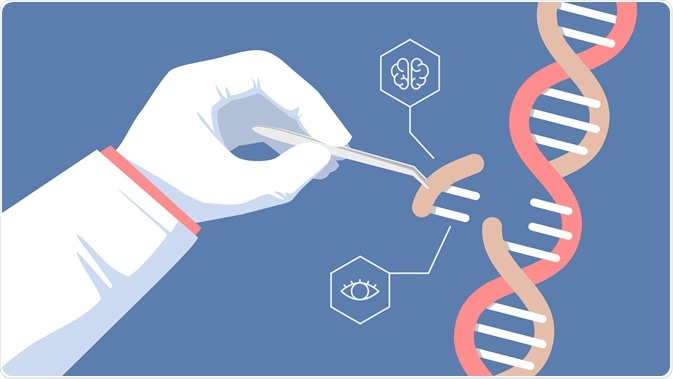
Image Credit: Panuwach/Shutterstock.com
It is based on the current knowledge of the mechanisms by which DNA damage is repaired, resulting in structural changes.
The meganuclease approach
Early on, targeted gene editing began with a meganuclease formed by fusing the catalytically active domain of the Fokl protein (a restriction endonuclease) with an engineered I-Scel that could target sequences of 18 base pairs. The meganuclease is highly specific in its cleavage site.
Zinc finger nuclease
Pairs of zinc finger nucleases (ZFN), or ZFN dimers, were also used, comprising an array of ZF modules (each specific for a 3-nucleotide sequence of DNA) fused to a FokI nuclease domain to target a site of 36 base pairs.
ZFNs are smaller and more precise in their effects than CRISPR-based methods and can be diversified, but are not used nearly as commonly, as only one company holds the patents and has the expertise to make them.
TALENs
Transcription activator-like effector nucleases (TALENs) use the same platform but with ZF being substituted by 14 to 24 TALENs, each specific for a single base-pair. Their size is intermediate between that of Cas9 and ZFNs.
DNA breaks and repair
Using a pair of ZFN or TALEN fusion proteins, the double strand of DNA at the targeted site is broken at a specific sequence. This double-stranded break (DSB) triggers DNA repair mechanisms that are responsible for the targeted change.
DSB repair can occur via homology-directed repair (HDR) that depends on homologous DNA sequences. Exogenous homologous sequences can be inserted in this way.
On the other hand, nonhomologous end joining (NHEJ) can occur, which introduces changes as the broken ends are joined. These changes are called indels (insertions and deletions).
CRISPR-Cas9
Today, the most visible face of gene editing is CRISPR-Cas9 (clustered regularly interspaced short palindromic repeats-CRISPR-associated protein 9).
Here, the Cas9 enzyme, which is actually sourced from bacterial defenses against viruses, inserts DSBs at the DNA sites which 1) are complementary to a guide RNA (gRNA) and 2) have an adjacent protospacer motif (PAM) sequence.
The repair process knocks out the target sequence, or, via HDR, inserts a fragment of DNA into the genome at the rejoined ends.
Difficulties with gene-editing techniques
There are problems with all these platforms.
ZFs have limited targetable sequences and DNA redundancy. The creation and purchase of optimized ZFN modules are complex and expensive.
On the other hand, the creation of TALE modules and the endonuclease domain takes too much time. TALENs bind to DNA with high affinity and with low off-target effects, but their repeated sequences are difficult to clone.
It takes a hundred times as long to make a highly precise meganuclease, which can be customized to recognize the desired sequences, compared to a CRISPR array. Hybrid megaTALs have been used, adding the simplicity of assembly of the TALENS with the specificity of cleavage of the meganuclease.
CRISPR needs to use PAM which restricts the type of targetable sequences. It is too large for viral vectors to insert into cells. Off-target mutations are also a problem.
The current gene editors cannot be used to correct point mutations because the indels may cause unintended mutations.
Newer gene-editing technologies
Site-specific recombinases
Site-specific recombinases are one way to avoid unpredictable indels due to HDR. They do not rely on host factors to break and rejoin the strands of DNA or on exogenous DNA sequences. Their efficiency produces virtually complete recombination within a few minutes.
Moreover, they can even turn the orientation of the DNA segment completely around. This is important in making circuit-like pathways that can regulate the behavior of the cell.
However, their target sites are complex and rare, comprising 30-50 base pairs. They can be attached to specific ZF modules to create fusion proteins.
Or they can use a directed evolution technique to produce SSRs with other specific targets. SSR technology is still new and time-consuming, however.
CRISPR variants
A variant of Cas9 is also being used that makes single-strand cuts or no cuts at all. This technique uses Cas9 fused to transcription regulators, or to enzymes that modify the DNA or associated histones, causing altered gene expression.
This biomimetic epigenetic process avoids off-target mutations.
CRISPR continues to improve with the development of new gRNAs, and other Cas enzymes targeting other PAMs or targets. These include spCas9-NG, xCas9, base editing, Cpf1, Cas13, and Cas14.
Cas13 targets RNA for RNA base editing, and can direct DSB repair towards NHEJ rather than HDR. Cas9 can also be switched on or off by fusing it with small molecules to limit off-target mutations, making it a controllable system.
The RESCUE system (RNA Editing for Specific C to U Exchange) can edit twice as many mutations as earlier RNA editors and can multiplex C to U and A to I editing using customized gRNAs.
The xCas9 variant can recognize a broader range of PAMs, with more specific and efficient DNA recognition and editing.
Cpf1, unlike Cas9, creates staggered DSBs at the distal position of a PAM. This enhances NHEJ efficiency for gene insertion. It only needs a 42-nucleotide CRISPR-RNA compared to the 100-nucleotide gRNA for Cas9.
Cas9-NG recognizes a PAM with minimal NG and can be used to edit human cells.
CRISPR-Cas14a is capable of introducing cuts independent of the adjacent sequence and does not require a PAM near the target site.
A hairpin secondary structure engineered onto the spacer region of sgRNAs boosts the specificity of the CRISPR system by several orders of magnitudes, allowing still more precise cleavage.
Base editing
Another recent technique is base editing, which introduces precise changes in single base pairs and avoids off-target mutations because there is no DSB repair involved, and therefore no HDR or NHEJ, or need for donor DNA.
The first base pair editor to be developed comprised a disabled Cas9 fused to a deaminase that converts cytosine to uracil. The U-G base pair that results become A-T, as DNA replicates.
Thus, the fusion protein acts on a DNA sequence to change cytosine-guanine to thymine-adenine in the DNA sequence permanently, without DSBs and therefore with low error rates.
Another changes adenine-thymine to guanine-cytosine. These two systems are able to handle a third of all the base pair changes that can be made and correct almost two-thirds of harmful human mutations presently known.
In fact, more than 100 base editing experiments have been successfully completed, some correcting diseases caused by human point mutations in animal models.
Sources
- Tachibana, C. (2019). Beyond CRISPR: What’s current and upcoming in genome editing. www.sciencemag.org/.../beyond-crispr-what-s-current-and-upcoming-genome-editing
- Manghwar, H., Lindsey, K., Zhang, X., and Jin, S. CRISPR/Cas system: recent advances and future prospects for genome editing. Trends in Plant Science 2019. Volume 24, Issue 12, P1102-1125, https://doi.org/10.1016/j.tplants.2019.09.006. www.cell.com/trends/plant-science/fulltext/S1360-1385(19)30243-2
- Moon, S. B., Kim, D. Y., Ko, J.-H., and Kim, Y.-S. Recent advances in the CRISPR genome editing tool set. Experimental and Molecular Medicine 2019, 51, Article number130. https://doi.org/10.1038/s12276-019-0339-7. https://www.nature.com/articles/s12276-019-0339-7
- Offord, C. (2016). Advances in Genome Editing. www.the-scientist.com/news-opinion/advances-in-genome-editing-33678
Further Reading