Much of what is known about human genetics and growth, has been garnered through work on worms, flies, fish, mice, and frogs. Though vastly different from humans, these organisms share common anatomical features that develop via a conserved mechanism.
Drosophila, or fruit flies, have been extensively used as model organisms in developmental genomics. Providing the foundations to our understanding of vertebrate development, drosophila have informed our knowledge of embryonic asymmetry and generation of the body axis.
Evidence suggests much of the anatomical differences that arise between species can be attributed to differences in regulatory DNA. These sequences allow for the same proteins to form radically different cells, based on when in developmental time the gene is expressed.
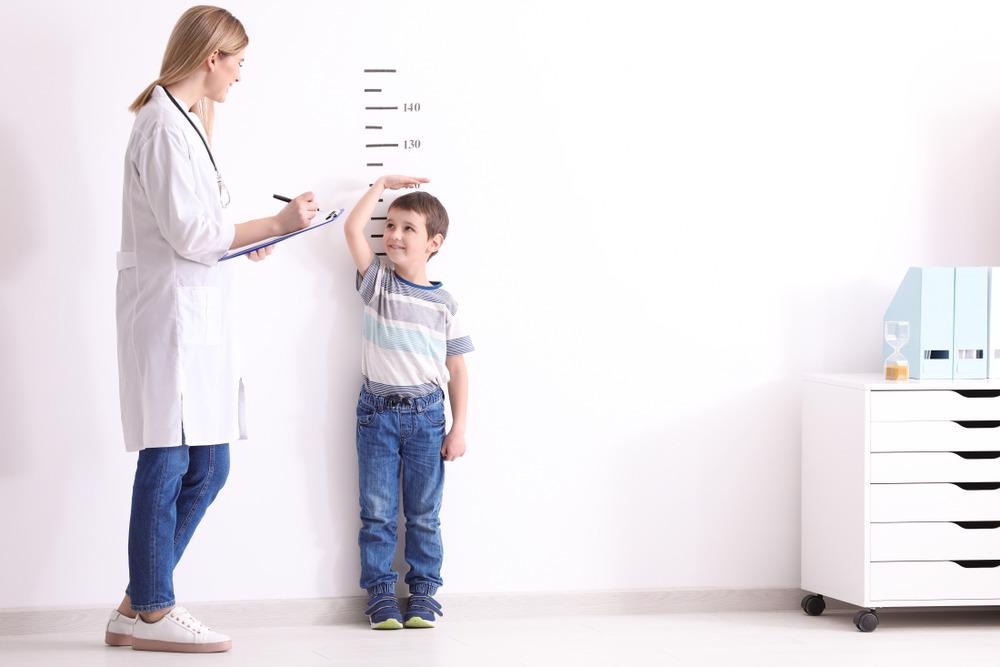
Image Credit: Africa Studio/Shutterstock.com
Cells of the embryo will become progressively more specialized
During the early stages of development, the fertilized egg rapidly divides and the maternal mRNA is degraded. This process activates the zygote genome and leads to the formation of a blastula. Identification of genes responsible for activating this stage of development has yet to be determined, but the DUX-family genes are current candidates. Mouse knock-out models show promising results, with impaired early embryonic development and defective genome activation.
In these early stages, the cells of the blastula are often totipotent or pluripotent. But, as morphogens are expressed in the zygote, the cells will become more specialized through the course of development. Spatiotemporal gradients of morphogens inform cells of their fate. Transforming growth factor-ß (TGF-ß), Wnt, Hedgehog, Notch, and receptor tyrosine kinase (RTK) pathways are critical signals in defining spatial patterns. These signals direct cells to new developmental pathways by combinatorial control and cell memory.
Establishing an axis and the primitive streak
Hox genes help to set up the anterior-posterior axis, with bone morphogenic protein (BMP) and its inhibitors defining the dorsal-ventral axis. Posteriorizing signals and their antagonists determine the germs layers of the zygote, in a process called gastrulation. The primitive streak forms early on in this process, in a region of high BMP, Wnt, FGF, and Nodal signaling; acting as a signaling hub at the posterior of the embryo.
Signaling gradients are set up by diffusion, with long-range inhibition and local positive feedback loops generating asymmetry and complex cellular patterns. SOX17 is a key transcription factor in defining the endoderm; the inner lining of the germ layers that creates a rudimentary gut in the embryo will give rise to epithelial cells. A network of transcription factors, including GATA2, GATA3, TFAP2A, and TFAP2C have been identified as defining the ectoderm. This forms the outer layer of the embryo and will give rise to the epidermis, as well as the neural tube. Moving between these two germ layers is the mesoderm.
An initially loose set of cells that will give rise to connective tissues, the mesoderm also forms the notochord and somite of the embryo. The notochord is a major source of signaling in coordinating the development, secreting fibroblast growth factor (FGF) and TGFß to inform correct patterning of the anterior mesoderm. Along with the neural tube, the somite and notochord form from the primitive streak. A hub from which the cells responsible for patterning of many organ systems emanate, the primitive streak and its derivatives are vital to development.
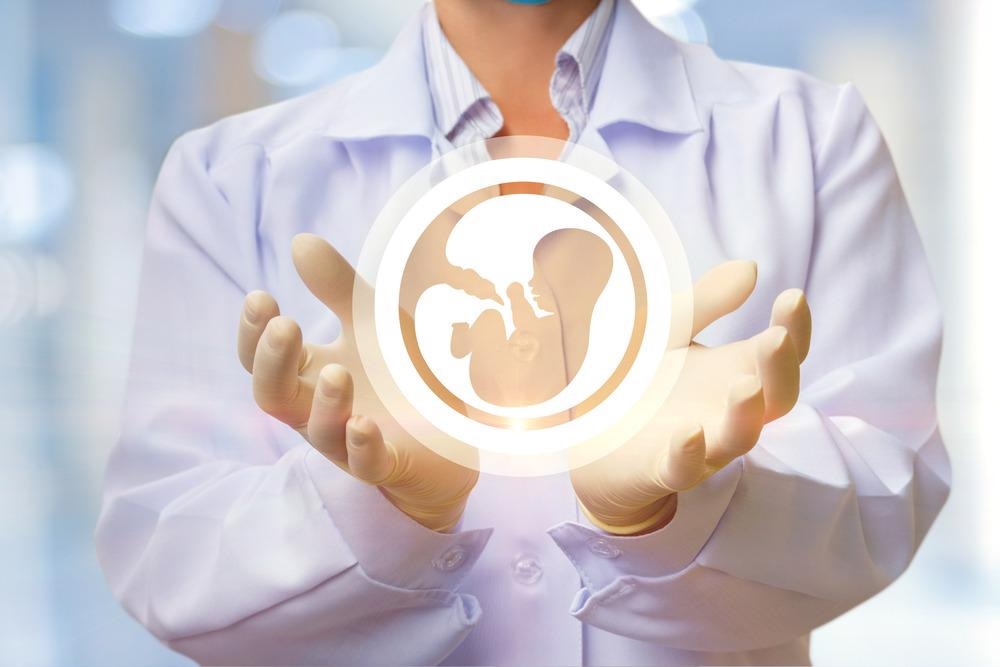
Image Credit: Natali _ Mis/Shutterstock.com
Defining fate maps in the embryo
Through the course of gastrulation, cells of the embryo undergo epithelial-to-mesenchymal transition (EMT) at the primitive streak. This first defining morphological event in this process is the breakdown of the basement membrane (BM) between the endoderm and mesoderm. Following removal of the BM, the primitive streak migrates distally and anteriorly to define cell fate maps.
The fate maps produced by proximity to primitive streaks are highly reproducible. As the primitive streak forms in a region of high signaling, cells that ingress early on are exposed to these high levels. Nodal activity remains restricted to the anterior of the embryo during gastrulation, as the primitive streak elongates. Determining cell lineages from posterior-to-anterior, the primitive streak produces gradients of signals that define the cell fates to a lateral mesoderm and definitive endoderm, respectively.
In the final stages of gastrulation, cells interact locally and by long-range diffusible signals to refine their fates. Cell fate is affected by when in developmental time the cell passes through the primitive streak, as well as their proximity. The location of the cell and the time it takes to arrive at its final location will also influence cell fate. During these early stages, the levels of signals impart detailed information for cell determination.
Sources:
- Bardot E.S., and Hadjantonakis A.K. (2020) Mouse gastrulation: Coordination of tissue patterning, specification and diversification of cell fate. Mech Dev. doi:10.1016/j.mod.2020.103617
- Ortega N.M., et al. (2018). Functional genetics of early human development. Curr. Opin. Genet. doi.org/10.1016/j.gde.2018.04.005.
- Alberts B., et al. (2015) Molecular Biology of the Cell, Sixth Edition. Int J Mol Sci. doi:10.3390/ijms161226074
Further Reading