Lab-grown organs, commonly known as organoids or mini-organs, are small, self-organizing structures developed in the laboratory to mimic the structure and function of human organs.1
While not yet fully functional or transplantable, these structures allow researchers to study organ development, model disease, and explore potential treatments.
This article explores the current state of lab-grown organ research, recent milestones, and ongoing clinical efforts at translating these advances into practical therapies.
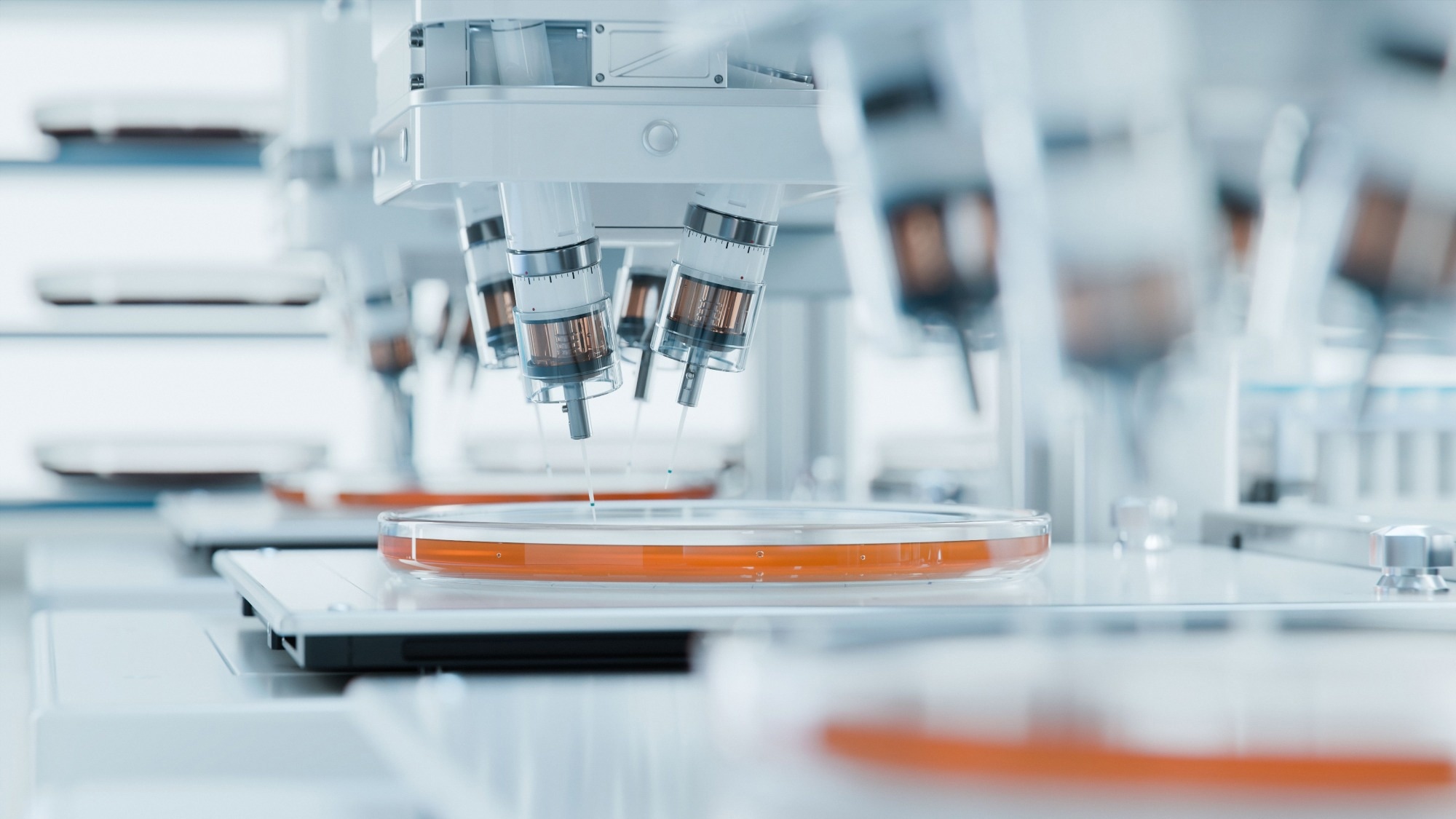
Image Credit: IM Imagery/Shutterstock.com
How Organoids Are Made?
Organoids are three‐dimensional (3D) miniaturized structures of organs or tissues developed from stem cells that possess self‐organizing and differentiating potential.
Scientists use advanced 3D culture technologies, along with specific growth factors and small-molecule inhibitors, to grow pluripotent stem cells (PSCs), adult stem cells (ASCs), embryonic stem cells (ESCs), and tumor cells in a 3D matrix. These cells then self-organize within the matrix to ultimately form organoids.2 Typically, these cells are obtained from healthy donors or patients.
PCSs are used to develop organoids because they can self-replicate and differentiate into any cell type. Scaffold-based or scaffold-free techniques are used to create organoid culture systems, which preserve the parental biological characteristics, gene expression, and mutation profiles of the original tissue in vitro.3
Organoids have now been successfully derived from a range of human organs and tissues, such as the lung, brain, heart, liver, and kidney. This has enabled new applications in large‐scale drug screening, toxicity testing, biobanking, drug discovery, biomarker identification, and precision medicine.4
More recently, by combining genome editing with 3D culture techniques, scientists have developed organoids to model hereditary diseases that were previously difficult to model in vitro. In the future, lab-grown tissues and, eventually, organs could play a role in treating patients with organ failures.
Download your PDF copy now!
3D Bioprinting for Lab-Grown Organs: Techniques and Challenges
3D bioprinting techniques are used to fabricate 3D bio-structures with precise geometric designs.5 This strategy has helped bridge the gap between synthetic and natural tissue constructs.
In 3D bioprinting, cells are deposited on a matrix known as bioink, which replicates the physicochemical environment of native tissue and supports cell adhesion, proliferation, and differentiation.
3D bioprinting has been crucial in scaffold construction, significantly improving microstructural detail and allowing for more anatomically accurate features.
Continued progress in developing novel biomaterial inks, which are highly hydrated polymeric networks, alongside cell biology-inspired computational design strategies, has helped researchers reach milestones in creating precise, tissue- or organ-like constructs with specific functionality.
Several bioprinting strategies have been developed, including laser-based methods, extrusion, dual channel, digital light processing (DLP), and stereolithography (SLA).6 These techniques have enabled the fabrication of various tissue structures, including bone, cartilage, dental, cardiovascular, cornea, neural, liver, glands, respiratory, urinary, and reproductive systems.
However, researchers still face major challenges in replicating the complexity of natural tissues and organs. Bioprinters must handle and print a variety of biomaterials alongside multiple cell types simultaneously to accurately construct functional structures. Selecting suitable bioinks is also essential for successful fabrication.
In 3D environments, interactions between cells and biomaterials influence cell proliferation, differentiation, and viability. As such, the surface chemistry, charge, reactivity, and hydrophilicity of the materials must be carefully considered.7 Therefore, despite advancements in 3D bioprinting, further research is needed before biofabricated constructs can be reliably used in clinical settings.
3D-Printing Heart Tissue With Human Stem Cells
So, How Far Off Are Lab-Grown Organs? Recent Progress and What’s Missing
While full organ fabrication remains out of reach, certain advances suggest that foundational elements of organ development are beginning to take shape in the lab.
Researchers at UC San Francisco and Cedars-Sina have recently developed a novel method to prompt stem cells to form specific organ structures.8
In typical lab-based organ development, cells from the placenta are often used to instruct stem cells to differentiate into more complex tissues. This new approach involved creating synthetic organizer cells that cluster near stem cells and send targeted growth signals to trigger their proliferation and differentiation.
Their research showed that a small number of organizer cells can be programmed to induce stem cells to form rudimentary, organ-like structures. These include constructs that contract like a beating heart and contain structures resembling ventricles.
This finding highlights the growing feasibility of creating human organs from scratch, which has been a long-term goal of researchers in regenerative medicine.
Using cellular reprogramming technology, scientists are now able to grow new organs and tissues from a patient’s own stem cells. Current efforts focus on enhancing the differentiation of stem cells to clinically useful cell types, alongside computational tools for assessing cell safety.
At present, fully functional organs have not yet been generated in laboratory settings. However, promising results have emerged from efforts to grow clusters of functional cells that can be transplanted into the body.
This approach may prove useful for treating specific conditions, such as repairing parts of the heart, nervous system, or bone marrow. In the case of diabetes, patients could potentially benefit from the implantation of lab-grown pancreatic islet cells.
Researchers are also exploring the use of organoid-derived tissue for transplantation. One ongoing clinical trial is assessing the safety and efficacy of using intestinal organoids to treat inflammatory bowel disease (IBD).9
Similarly, the Yokohama City University (YCU) Advanced Medical Research Center, in collaboration with other universities in Japan and the USA, is working on organoid-guided precision hepatology for treating metabolic liver disease.10
The Center for Stem Cell and Organoid Medicine (CuSTOM) has also developed a platform for generating patient-derived induced pluripotent stem cells (iPSCs), supporting the acceleration of organoid-based therapies.11
These are just a few examples of how the field is moving from basic research toward real-world clinical applications. For recent updates, publications, and ongoing research in this area, visit:
References and Further Reading
- Yang, S., et al. (2020). Organoids: The current status and biomedical applications. MedComm.. doi: 10.1002/mco2.274.
- Heinzelmann, E., et al. (2024). iPSC-derived and Patient-Derived Organoids: Applications and challenges in scalability and reproducibility as pre-clinical models. Curr Res Toxicol. . doi: 10.1016/j.crtox.2024.100197.
- Liu, X., et al. (2025). Recent progress on the organoids: Techniques, advantages and applications. Biomed Pharmacother. doi: 10.1016/j.biopha.2025.117942.
- Zhou, Z., et al. (2021). Patient-Derived Organoids in Precision Medicine: Drug Screening, Organoid-on-a-Chip and Living Organoid Biobank. Front Oncol. . doi: 10.3389/fonc.2021.762184.
- Wu, CA., et al. (2023). Advances in 3D Bioprinting: Techniques, Applications, and Future Directions for Cardiac Tissue Engineering. Bioengineering (Basel). doi: 10.3390/bioengineering10070842.
- Loukelis, K., et al. (2024). Advances in 3D bioprinting for regenerative medicine applications. Regen Biomater. doi: 10.1093/rb/rbae033.
- Gungor-Ozkerim, PS., et al. (2018). Bioinks for 3D bioprinting: an overview. Biomater Sci. doi: 10.1039/c7bm00765e.
- Yamada, T., et al. (2025). Synthetic organizer cells guide development via spatial and biochemical instructions. Cell. doi: 10.1016/j.cell.2024.11.017.
- Hammerhøj, A., et al. Organoids as regenerative medicine for inflammatory bowel disease. iScience. 2024;27(6):110118. doi: 10.1016/j.isci.2024.110118.
- Osonoi, S., Takebe, T. (2024). Organoid-guided precision hepatology for metabolic liver disease. J Hepatol. doi: 10.1016/j.jhep.2024.01.002.
- Takebe, T, et al. (2018). Organoid Center Strategies for Accelerating Clinical Translation. Cell Stem Cell. doi: 10.1016/j.stem.2018.05.008.