There have been extensive efforts to develop alternative drug delivery strategies, particularly for peptides like insulin used to treat endocrine disorders, specifically diabetes. This is because protein and peptide therapeutics require parenteral (delivery by the intravenous route) administration, which serves as a barrier or deterrent to adherence to medication regimes. Oral delivery is therefore desirable, but it is challenged by several barriers related to the organization and physiological function of the gastrointestinal tract.
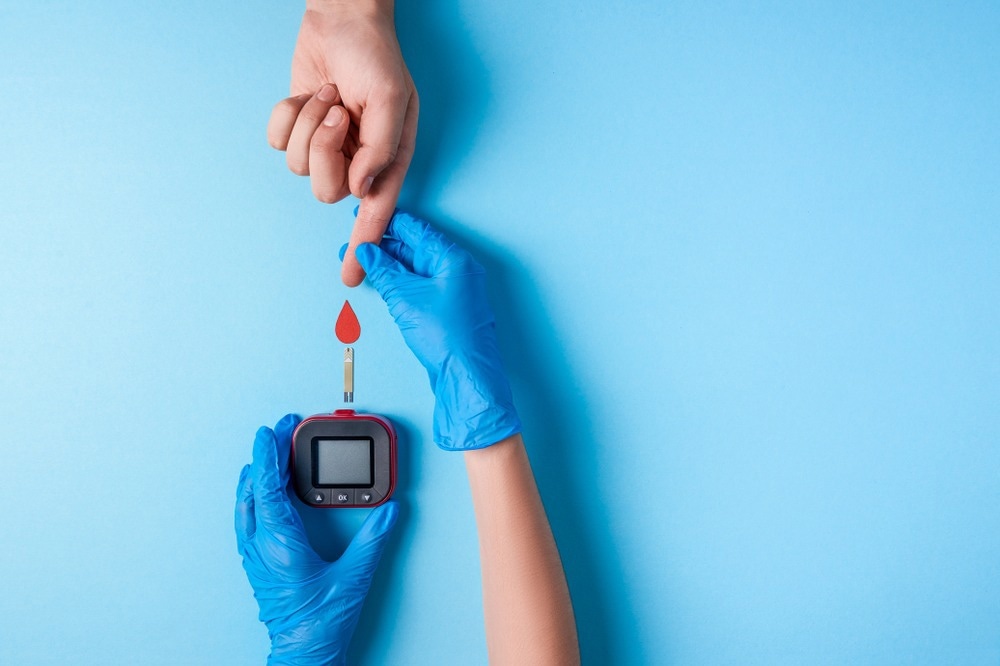
Image Credit: Kateryna Novikova/Shutterstock.com
Why Is There a Need for Oral Routes of Administration in Diabetes?
Oral therapeutics for diabetes sit under the umbrella term of biologic therapeutics, which include large proteins, antibodies, hybrid fusion proteins, therapeutic peptides common tie, and antibody and drug conjugates. These therapies are administered via injection as they are poorly bioavailable via the oral cavity.
Consequently, research has explored the feasibility of delivering proteins via alternative routes with oral, nasal, pulmonary, buccal, ophthalmic, and transdermal administration being considered. Oral drop delivery is particularly attractive for patients due to its widespread use and convenience. However, the use of oral therapeutics is hampered by the anatomical structure and function of the gastrointestinal system. The challenges to efficient oral absorption of peptides are as follows:
- The mucus barrier: the gastrointestinal tract has evolved a lining complete with a sophisticated set of cellular and mucosal barriers to restrict the access of enteral microbiota, toxic agents, and pathogens
- Gastrointestinal enzymes
- Protein lumenal export or degradation
- Intracellular environment
What Approaches Have Been Developed to Overcome the Permeability Barrier for Oral Therapeutics for Diabetes?
Paracellular approaches are designed to facilitate the movement of a peptide-based drug through transient disruption of cellular junctions. In contrast, transcellular approaches enable the movement of peptide drugs through the cytoplasm of enterocytes, which are secreted into the lymphatic or systemic circulation.
Technologies that have been employed for the absorption of peptides along different regions of the gastrointestinal tract Among these strategies, the most notable types include permeation enhancers; and a permeation enhancer has recently been used in the approved oral formulation of semaglutide –an anti-diabetic medication used for the treatment of type 2 diabetes.
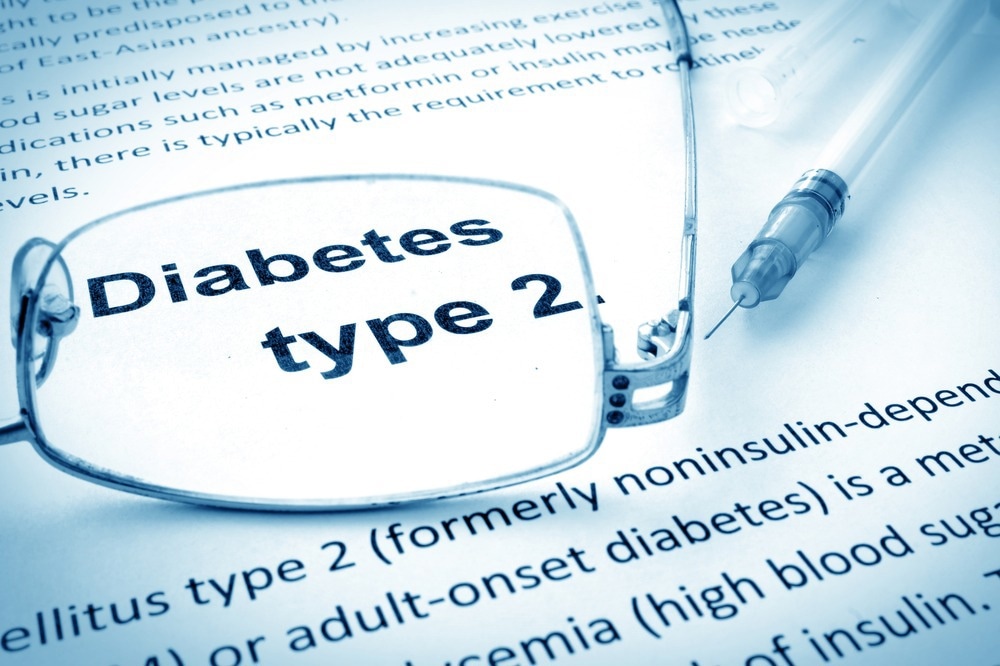
Image Credit: Vitalii Vodolazskyi/Shutterstock.com
Permeation Enhancers
Permeation enhancers the target of the transcellular route by facilitating the movement of non-degraded peptides through the epithelial cells. Alternatively, permeation enhancers operate via the paracellular route, interfering with the intercellular junction adhesion proteins.
Enhancers must be able to transiently disrupt the intestinal epithelium to enable peptide absorption while ensuring an acceptable safety profile without any local minimal or systemic toxicity. Permeation enhancers with different modes of action can be combined at low doses to minimize cell toxicity while still working synergistically to enhance permeation's efficacy. Among them, very few have shown sufficient efficacy and safety to be able to progress from preclinical to clinical trials.
Two permeation enhancers, sodium caprate and sodium N-caprylate (also known as SNAC) are examples of two that have been used in proprietary delivery platforms for several years. Sodium caprate is a medium-chain fatty acid that occupies an ionized soluble says and shows detergent capacity at the pH value of the small intestine. It affects both the transcellular and paracellular roots. SNAC is classified as "generally recognized as safe" (GRAS) and operates predominantly via the transcellular mode of transit through the epithelium.
There are several concerns associated with permeation enhancers, namely the potential for the increased likelihood that noxious agents, including pathogenic organisms, will penetrate the barrier, along with the potential for the development of immune responses or disruption to the gut microbiome. As of yet, no such toxicities have emerged; for example, the largest trial to date, which examines the safety of oral insulin, has demonstrated that when subjects are treated with an insulin analog encapsulated with sodium caprate.
Only a small number of patients developed human anti-insulin and cross-reacting antibodies. This, therefore, precluded any significant conclusions about the significance of these antibodies from being drawn. Alongside this, the largest trial of oral semaglutide, which examined the long-term cardiovascular safety of oral semaglutide, co-formulated with SNAC, demonstrated no serious adverse events.
Modulation of pH
The low pH of the stomach presents a significant barrier to the oral delivery of peptides. However, tablets that contain oral therapeutics for delivery can be coated with an acid-stable enteric coat. This thereby prevents them from dissolving in the stomach. After leaving the stomach, the oral therapeutic reaches the upper intestine, where the elevation in pH enables dissolution of the enteric coat, with subsequent release of the tablet contents. This approach has been used to develop an oral formulation for calcitonin, a treatment for Paget's disease of bone or hypercalcemia of malignancy.
To overcome the degradation by intestinal and pancreatic enzymes, citric acid is included in the tablet to produce a local transient decrease in pH. This is because the optimal pH for these gastrointestinal enzymes is neutral to basic, so citric acid inhibits resident peptidases. The coadministration of citric acid can also achieve this with oral formulations to enhance absorption.
Direct Enzyme Inhibition
Enzyme inhibition can circumvent the barrier of got enzyme activity. Inhibitors include aprotinin, soybean trypsin inhibitor, and leupeptin. The most clinically advanced example of this direct enzyme inhibition mean of administration is an oral insulin formulation which includes a soybean trypsin inhibitor and a curating agent that scavenges calcium. This functions to sequester calcium, a cofactor from a protease. Despite this approach being diploid, the utility and safety of enzyme inhibition as a general strategy for improving the absorption of oral peptides are unknown.
Peptide Cyclization
Cyclization strategies remove exposed termini from the N and si ends of peptides particularly vulnerable to cleavage by enzymes. A cyclic structure is traditionally a naturally occurring feature of small peptides. There have been two successfully developed for oral administration; the immunomodulatory drug cyclosporin and desmopressin, which is an analog of the peptide hormone vasopressin, which exhibits greater resistance to degradation relative to vasopressin. Despite this, the extent of its feasibility as a generalizable strategy for oral peptide absorption is not promising. The majority showed limited oral bioavailability in a study of physical-chemical parameters and oral absorption properties of 125 cyclic peptides.
Other strategies include:
- Mucus-penetrating agents: This enables the drug to get past the mucus barrier. In the context of oral therapeutics for diabetes, insulin delivery in preclinical studies has been achieved through the development of cell-penetrating peptide (CPP)–insulin conjugates encapsulated in mucoadhesive N-trimethyl chitosan chloride-coated PLGA nanoparticles. However, safety and efficacy remain to be validated in large clinical trials
- Cell-penetrating peptides (CPPs) are generally efficient at translocating the membrane as they are derived from viruses that can do this. Alternatively, they may be non-viral proteins or smaller molecules. CPPs can interact with membrane components such as amino glycans and traverse endocytotic pathways whereby they can deliver their protein cargo into systemic circulation via exocytosis. Energy-dependent and energy-independent pathway mechanisms have been identified as facilitators of CPP and cargo entry. Although they have been explored for anti-cancer and antimicrobial therapy, their utility in enhancing oral absorption of peptide therapies, like diabetic therapy, has not yet been validated in the clinical setting.
- Intestinal patches enable a small reservoir of the drug to be physically protected from local degradation and position the drug close to the epithelium, where it is absorbed. An example of this is a mucoadhesive patch capable of adhering to the small intestine mucosa in pigs and rats, where it was shown to release calcitonin, which was detected in systemic circulation and achieved its indication of reducing blood calcium. More recently, an adhesive patch that disrupts intestinal tight junctions to facilitate drug transport via the paracellular route has been reported in the context of insulin delivery into systemic circulation in rats via electric current-mediated entry and did not show evidence of structural impairments to the gut mucosa. Although promising in preclinical studies, chemical proof of concept is yet to be demonstrated
- Hydrogels: these contain water across linked polymer and a peptide-based cargo drug, alongside mucoadhesive polymers. Together these facilitate prolonged retention and increase the time that the peptide resides in the gut while providing resistance to enzymatic degradation
- Microneedle devices and mini-posts: these encompass device-based delivery technologies. An example of this is an enteric-coated capsule for the delivery of biological therapeutics, including peptides such as insulin. The capsule undergoes pH-dependant dissolution in the small intestine, with the concomitant release of carbon dioxide used to inflate a small balloon that positions a microneedle-based device next to the epithelium. This enables the injection of the therapeutic cargo through the mucosa. Another two technologies include a luminal unfolding microneedle injector (LUMI) and an orally ingested self-orienting millimeter-scale applicator (SOMA). LUMI includes poly(methacrylic acid-co-ethyl acrylate) and PEG coating of multiple drug-loaded microneedles; the coating dissolves at the pH of the small intestine. SOMA is capable of adhering to the gastric mucosa and delivers pharmaceutical products such as insulin via injection. This does not require puncturing the outer layer of the stomach
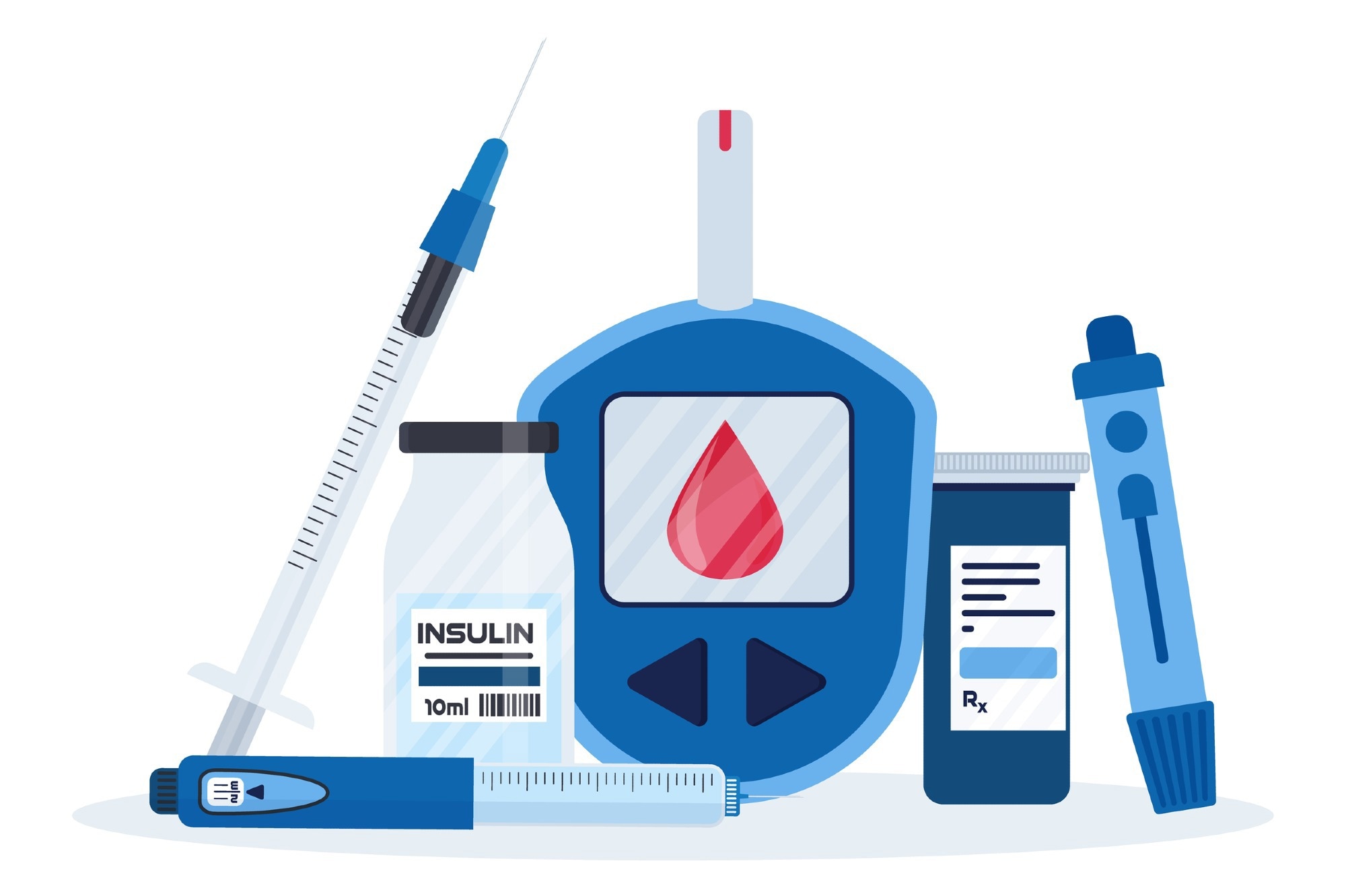
Image Credit: Svetolk/Shutterstock.com
The Current State of Oral Therapeutics for Diabetes
Insulin itself has been formulated with several permeation enhancers for oral delivery. SNAC has not been as high concentrations of SNAC can interfere with the glucose drink property of insulin. By contrast, sodium caprate has been extensively studied; for example, a long-acting insulin analog delivered in a tablet containing sodium caprate for once-daily administration was compared against a once-daily subcutaneous injection of insulin, insulin glargine, over 8 weeks elect phase two trial of 50 patients with type 2 diabetes.
The primary endpoint, which was reductions in fasting glucose, was similar in both treatment arms, and the rates of adverse events what's similar between both groups. Despite the promising results, mechanical development of the permeation-enhanced insulin was discontinued due to low bioavailability (between 1.5 and 2%).
Another oral form of oral therapeutics for diabetes is an insulin analog formulated with sodium caprate, which contains a methoxy-trimethylene-glycol-propionyl moiety linked to the Lys-β29 amino group formulated to act quickly to reduce postprandial hyperglycemia. Dose proportional increases in plasma insulin and reductions in blood glucose following administration of 10 to 30mg tablets were found.
In addition to permeation enhancers and protein delivery strategies, insulin-containing nanoparticles have been used for oral therapeutics for diabetes. These nano-articles showed greater epithelial uptake and are less likely to be targeted to lysosomes for intracellular degradation.
Moreover, insulin nanoparticles undergo enhanced transmucosal passage relative to the non-modified insulin. 'Bubble' carriers composed of diethylenetriamine pentetic acid dianhydride and sodium bicarbonate can incorporate insulin in the form of bubbles.
Sodium dodecyl sulfate, also included in the bubble carriers, increases the dispersion of insulin molecules, stabilizes the carriers, and acts as both an inhibitor of proteases and enhances permeation. The paracellular transport of insulin has been enhanced when insulin preparations have been formulated with choline; this also reduces enzymatic incineration and decreases the thickness of the gastrointestinal mucus layer to enhance bioavailability.
Most current oral therapeutics for diabetes target the stomach and small intestine at present. However, the colon has been viewed as a favorable region to YouTube faster rates of transit, a pH closer to neutral, decreased levels of decorative enzymes and increased susceptibility to permeation enhancers. To achieve this colonic targeting, insulin has recently been co-formulated with modified nanoparticles, amphipathic chitosan derivatives, and a series of CPPs. These facilitate transcellular transmucosal passage.
Despite the promise and attractiveness of oral therapeutics for diabetes, there are still several bioavailability roadblocks in oral insulin delivery systems. This is coupled with a high cost of manufacturing recombinant insulin, which would mean that competitive price marketing oral therapeutics for diabetes could prove challenging. The discontinuation of permeation-enhanced insulin has illustrated this.
Sources:
- Drucker DJ. (2020) Advances in oral peptide therapeutics. Nat Rev Drug Discov. doi: 10.1038/s41573-019-0053-0.
- Banerjee A, Ibsen K, Brown T, et al. (2018) Ionic liquids for oral insulin delivery. Proc Natl Acad Sci U S A. doi: 10.1073/pnas.1722338115.
- Eldor R, Arbit E, Corcos A & Kidron M. (2013) Glucose-reducing effect of the ORMD-0801 oral insulin preparation in patients with uncontrolled type 1 diabetes: a pilot study. PLOS ONE.
- Geho WB, Rosenberg LN, Schwartz SL, et al. (2014) A single-blind, placebo-controlled, dose-ranging trial of oral hepatic-directed vesicle insulin add-on to oral anti-diabetic treatment in patients with type 2 diabetes mellitus. J Diabetes Sci Technol. doi: 10.1177/1932296814524871.
- Wu S, Bin W, Tu B, et al. (2014) A Delivery System for Oral Administration of Proteins/Peptides Through Bile Acid Transport Channels. J Pharm Sci. doi: 10.1016/j.xphs.2019.01.027.
- Guo F, Ouyang T, Peng T, et al. (2019) Enhanced oral absorption of insulin using colon-specific nanoparticles co-modified with amphiphilic chitosan derivatives and cell-penetrating peptides. Biomater Sci. doi: 10.1039/c8bm01485j.
Further Reading