According to the World Health Organization (WHO), the problem of increasing antibiotic resistance is one of the world’s biggest threats to health, food security, and development. While antibiotic resistance can occur naturally, the prevalence of resistance is being propagated by the widespread misuse of antibiotics around the world.
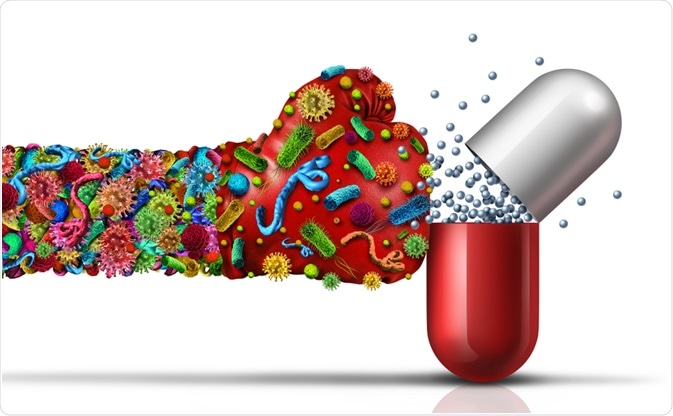
Image Credit: Lightspring/Shutterstock.com
As a result of improper use of antibiotics, some infections that were once easily controlled by antibiotics are becoming harder to treat as antibiotics have an increasingly reduced impact. Gonorrhea, pneumonia, salmonellosis, and tuberculosis are all becoming more difficult to treat due to increasing antibiotic resistance. Healthcare systems around the world are beginning to feel the pressure of this as well as the longer hospital stays and increased mortality associated with harder-to-treat infections.
Gaining a better understanding of how antibiotic resistance is established is vital to developing strategies to prevent it. Studies that reveal the nature of antibiotic resistance at the cellular level are instrumental in understanding the resistance mechanisms of the bacterial cell wall and other cellular structures that lead to antibiotic resistance. Here we discuss the current knowledge in this area.
The structure of the bacterial cell
Bacteria that cause infections are defined as being either Gram-positive or Gram-negative. Gram-positive bacteria have a tough and rigid cell wall that surrounds a cytoplasmic membrane. In contrast, Gram-negative bacteria have a lipid membrane called outer membrane (OM) that surrounds a thin cell wall.
The bacterial periplasm is the space situated in-between the OM and cytoplasmic membrane. The OM acts as an extra layer to protect Gram-negative bacteria from substances attempting to enter into the bacterium.
For many years, scientists have been developing antibiotics to compromise the structural integrity of the cell to induce lysis of bacterium. For decades, antibiotics have effectively served their purpose, but now, mostly due to misuse of these pharmaceuticals, bacteria that cause serious infection are becoming increasingly resistant.
How bacterial cell structure is linked with antibiotic resistance
The OM which protects bacteria from invading molecules also offers an opportunity for therapeutic defense against infections. The membrane layer contains porins, channels that allow molecules to pass through them. Scientists have developed therapeutic molecules that take advantage of the bacterium’s natural structure.
Drug molecules, such as antibiotics, can be taken up through the membrane via diffusion or by self-uptake. Hydrophilic molecules can cross the bacterium’s OM via the porins. A reduction in the number of porin channels is thought to underly increased resistance to certain classes of antibiotics, including β-lactam antibiotics.
Another way in which bacteria can reduce the efficacy of antibiotics is via the recruitment of membrane proteins known as efflux pumps. These pumps remove antibiotic molecules from the cell and maintain low intracellular concentrations by actively pumping out antimicrobial substances while they are moving into the cell.
Efflux pumps are situated in the cytoplasmic membrane, rather than in the OM-like porins. Most efflux pumps are multi-drug transporters that can pump out a wide range of antibiotics. All classes of antibiotics can be impacted by efflux pumps, and, therefore, antibiotic resistance can occur for any kind of antibiotic via the efflux pump system.
Modification of target molecules is another route to antibiotic resistance. The target sites of antimicrobials are subject to natural variations and acquired changes that result in the prevention of the drug-binding successfully with the target site. A spontaneous mutation is often the cause of changes to the structure of the target site. Even minor alterations to the site can lead to a significant reduction in the efficacy of an antibiotic due to the precise engineering of the drug to target specific sites.
Numerous common alterations can occur which lead to antibiotic resistance, here we review those that are most common.
Alterations that occur to the 30S subunit or 50S subunit of the ribosome causes resistance to antibiotics that target protein synthesis, including aminoglycosides, chloramphenicol, macrolides, tetracycline, and chloramphenicol.
Antibiotic resistance in Gram-positive bacteria is often the result of penicillin-binding protein modification. Enterococcus faecium resistance to ampicillin occurs via this pathway. Streptococcus pneumoniae resistance to both oxacillin and methicillin occurs via a similar route, with studies showing that integration of “staphylococcal cassette chromosome mec”, a genetic element, into the S.aureus chromosome, results in antibiotic resistance.
The bacterial strains of E. faecium and Enterococcus faecalis have developed high levels of resistance to teicoplanin and vancomycin via the process of cell wall precursor alterations in Gram-positive bacteria.
Other routes to antibiotic resistance are related to the modification of more than one molecule. An example of this is the modification of the DNA gyrase and topoisomerase IV enzymes which leads to fluoroquinolone resistance, causing DNA replication failure and resulting in the inability of fluoroquinolone to bind.
Finally, mutations to RNA polymerase have been shown to result in resistance to rifampicin.
Overall, there are a wide range of genetic mutations that can lead to alternations in cell structure, resulting in antibiotic resistance. Different antibiotics are often threatened by different types of mutations. Understanding more about these mutations at the level of the cell is vital to helping to develop antibiotics with higher levels of resistance to ensure that infectious diseases can continue to be combatted with highly effective drugs.
Sources:
- Antibiotic resistance. The World Health Organization. Available at: https://www.who.int/news-room/fact-sheets/detail/antibiotic-resistance
- Kapoor, G., Saigal, S., and Elongavan, A., 2017. Action and resistance mechanisms of antibiotics: A guide for clinicians. Journal of Anaesthesiology Clinical Pharmacology, 33(3), p.300. https://www.ncbi.nlm.nih.gov/pmc/articles/PMC5672523/
- Nikolaidis, I., Favini-Stabile, S. and Dessen, A., 2014. Resistance to antibiotics targeted to the bacterial cell wall. Protein Science, 23(3), pp.243-259. https://www.ncbi.nlm.nih.gov/pmc/articles/PMC3945833/
Further Reading