By Dr. Said QabbaahReviewed by Lexie Corner
Gut organoids are three-dimensional (3D), stem cell-derived structures that mimic the cellular composition and functions of the human gastrointestinal (GI) tract. By replicating digestive physiology in vitro, these models are increasingly used in research to study GI health and disease.1
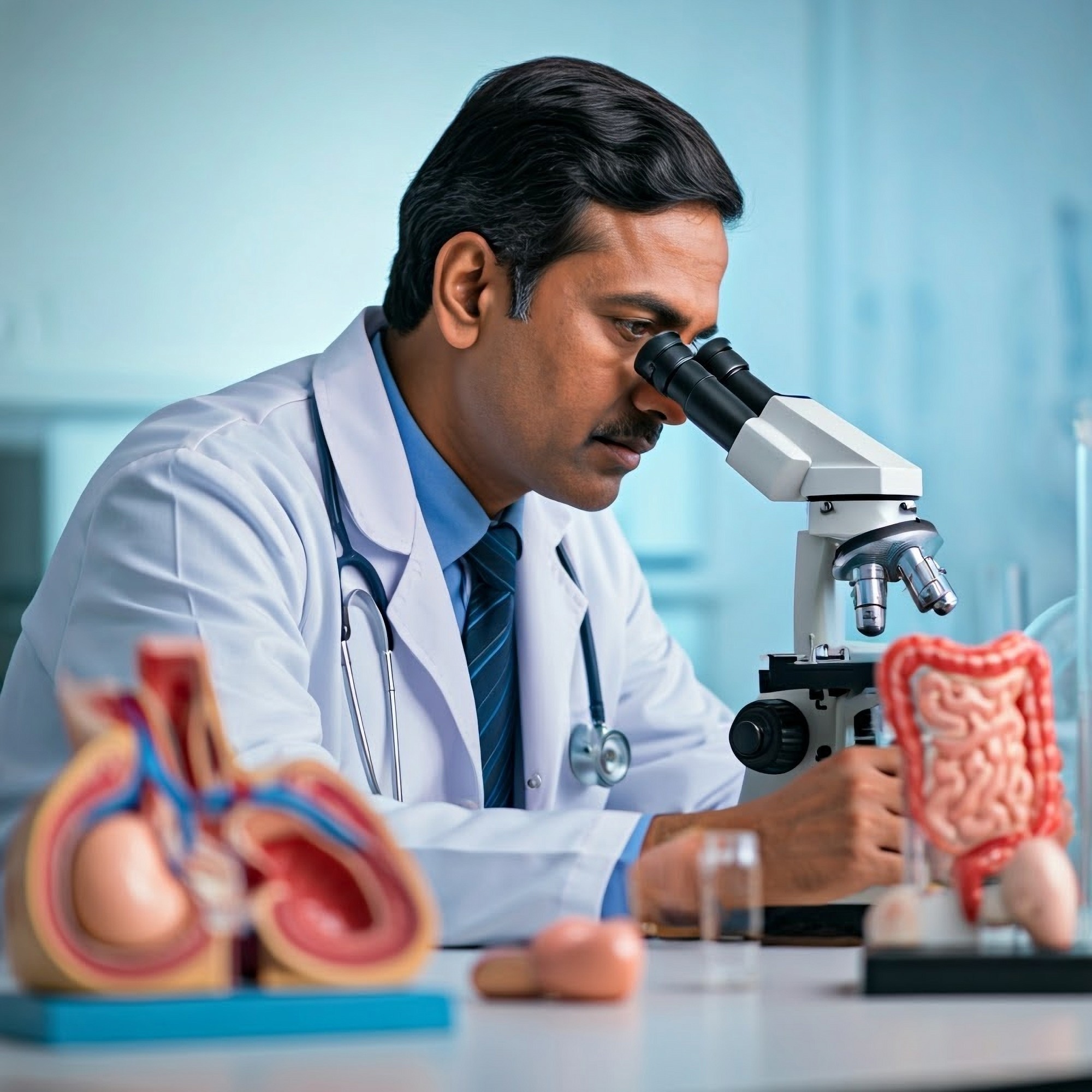
Image Credit: Shutterstock AI/Shutterstock.com
Modeling Digestive Regions with Gut Organoids
Gut Organoids in Action
Gut organoids offer a controlled system for modeling distinct regions of the human digestive system, including the stomach, small intestine, and colon.
Gastric Organoids
Gastric organoids model the structure and function of the human stomach, incorporating specialized epithelial cell types such as mucous, chief, endocrine, and parietal cells.2
These models enable the in-depth study of various gastric processes, such as acid secretion, mucosal barrier function, motility, lineage differentiation, and hormone regulation. In particular, they can mimic specific cell types in the fundus that secrete ghrelin, a hormone involved in appetite regulation and energy homeostasis, facilitating detailed investigation of metabolic pathways.2
They also support disease modeling for gastric pathologies, including peptic ulcer disease, Helicobacter pylori (H. pylori) infection, gastroparesis, and gastric carcinogenesis.2,3
Download your PDF copy now!
Small Intestinal Organoids
Small intestinal organoids closely resemble the crypt-villus architecture and cellular composition of native tissue, containing enterocytes, goblet cells, Paneth cells, and enteroendocrine cells.4
They replicate the absorptive and secretory functions of the small intestine, providing a platform to study nutrient absorption, hormone secretion, host–microbe interactions, and pathologies (e.g., celiac disease).4,5
Enteroendocrine cells within these organoids can secrete different hormones, including Glucagon-Like Peptide-1 (GLP-1) and Glucose-Dependent Insulinotropic Polypeptide (GIP), in response to luminal stimuli, allowing the study of postprandial hormone regulation.5
These models can also express brush-border enzymes (e.g., sucrase-isomaltase) and functional nutrient transporters (e.g., Sodium-Glucose Linked Transporter 1 (SGLT1), Peptide Transporter 1 (PEPT1)), enabling modeling of digestion and absorption.5
Colonic Organoids
Colonic organoids replicate the epithelial makeup of the colon, primarily including colonocytes, goblet cells, and other differentiated epithelial types.
They are used to study key functions such as barrier integrity, absorption, and immune–epithelial interactions. These models also support research into disease mechanisms and drug responses in conditions like inflammatory bowel disease and colorectal cancer.6
Their suitability for genetic modification makes them useful for exploring gene-specific effects and advancing precision medicine.6
For a more detailed look at how intestinal organoid cultures are transforming gut research, watch:
Intestinal Organoid Culture: The Next Dimension in Intestinal Research
Studying Enteroendocrine Cells and Nutrient Sensing
Hormonal Insights from Organoids
Gut organoids provide a platform for studying rare enteroendocrine cells, which are key regulators of gut–brain communication and metabolic processes.5
By exposing these models to different nutrient conditions, researchers can assess how factors like glucose or amino acid availability influence hormone secretion, including GLP-1, ghrelin, and peptide YY (PYY)—all of which affect appetite, glucose regulation, and GI motility.5
Modulating pathways such as mTOR (mechanistic target of rapamycin) and AMPK (AMP-activated protein kinase) further allows investigation into how enteroendocrine cells interpret nutrient signals to influence metabolic function.7
Advanced Organoid Techniques
One key technique for studying enteroendocrine cells in organoids is the induction of Neurogenin-3 (Ngn3), a transcription factor that directs their differentiation from gut stem cells. By adjusting Ngn3 expression, researchers can generate specific cell subtypes—such as enterochromaffin, L, and K cells—to investigate lineage development, nutrient sensing, and hormone signaling.5,7
Another approach involves Clustered Regularly Interspaced Short Palindromic Repeats (CRISPR)-based receptor screening in organoid cultures. This allows scientists to pinpoint receptors essential for nutrient detection and metabolic signaling.2,8
Targeted disruption of specific receptors or signaling molecules in organoid-derived enteroendocrine cells enables precise functional analysis of these pathways, advancing understanding of gut–metabolism interactions.8
Therapeutic Applications of Gut Organoids
Gut organoids offer several advantages for therapeutic development. They allow researchers to predict individual drug responses, helping tailor personalized treatments that improve outcomes and minimize side effects.1,3,6
Their capacity to support high-throughput screening of drug candidates enables assessment of efficacy and safety in a human-specific context, accelerating drug discovery and identifying more effective therapeutic targets.2,4
These applications extend to metabolic and motility disorders (e.g., obesity, type 2 diabetes, irritable bowel syndrome, gastroparesis), as well as GI cancers and other digestive conditions.5,6
They also enable studies of gut tissue regeneration, aiding the repair of damage from certain conditions (e.g., Crohn’s disease) and supporting the development of tissue replacement therapies.9
Challenges in Gut Organoid Research
Despite advancements in organoid technology, several challenges limit its broader application in research and clinical settings.
Reproducibility is a particular limitation, as variability in culture conditions and biological sources can cause inconsistent results. This is further complicated by the difficulty of scaling production to meet the needs of large-scale screening or clinical trials.4
Additionally, gut organoids often lack the full diversity of cell types found in the GI tract, such as smooth muscle, endothelial, and stromal cells, limiting their ability to model the digestive system’s complexity.4,10
The absence of key immune components (e.g., T cells, macrophages) limits their applicability in studying the immune system’s role in gut health and reduces their effectiveness for investigating conditions such as IBD, colorectal cancer, and GI infections.4
Future Directions in Gut Organoid Research
Recent advances in gut organoid research have improved the physiological relevance of these models, particularly in replicating complex tissue organization, dynamic environmental conditions, and host-microbe interactions.10
A major advancement is the development of organoid-on-chip platforms, which create controlled microenvironments that closely mimic in vivo conditions. By integrating microfluidics with organoid cultures, these systems allow precise control of key physiological parameters, enhancing functional gut-like behavior.2,10
Co-culture approaches add complexity to organoid models by incorporating additional cell types, such as mesenchymal, immune, and endothelial cells, to better replicate the cellular diversity and interactions of native tissue.4,10
Introducing microbiota into gut organoids further enhances co-culture techniques, enabling detailed analysis of the microbiome's role in gut health, and supporting the development of effective microbiota-based therapies.10
As these models become more sophisticated, they are increasingly useful for disease modeling, drug screening, and personalized medicine. Continued innovation will improve accessibility, scalability, and physiological relevance, positioning gut organoids as powerful tools for advancing gastrointestinal research and clinical applications.
Related Reading
Explore more on gut physiology and microbiome research:
References and Further Reading
- Kuhn, M.R., Wolcott, E.A., & Langer, E.M. (2025). Developments in gastrointestinal organoid cultures to recapitulate tissue environments. Frontiers in Bioengineering and Biotechnology, 13:1521044. doi: 10.3389/fbioe.2025.1521044
- Lee, S., Choi, J., Park, S., & Kim, J. (2024). Gastric Organoid, a Promising Modeling for Gastric Stem Cell Homeostasis and Therapeutic Application. International Journal of Stem Cells, 17:337-346. doi: 10.15283/ijsc23075. https://pubmed.ncbi.nlm.nih.gov/38698632/
- Seidlitz, T., Koo, B.K., & Stange, D.E. (2021). Gastric organoids—an in vitro model system for the study of gastric development and road to personalized medicine. Cell Death & Differentiation, 28:68–83. doi: 10.1038/s41418-020-00662-2. https://www.nature.com/articles/s41418-020-00662-2
- Taelman, J., Diaz, M., & Guiu, J. (2022). Human Intestinal Organoids: Promise and Challenge. Frontiers in Cell and Developmental Biology, 10. doi: 10.3389/fcell.2022.854740. https://www.frontiersin.org/journals/cell-and-developmental-biology/articles/10.3389/fcell.2022.854740/full
- Smith, C.A., Lu, V.B., Bakar, R.B., Miedzybrodzka, E., Davison, A., Goldspink, D., Reimann, F., & Gribble, F.M. (2024). Single-cell transcriptomics of human organoid-derived enteroendocrine cell populations from the small intestine. The Journal of Physiology. doi: 10.1113/JP287463. https://pubmed.ncbi.nlm.nih.gov/39639676/
- Munro, M.J., Tan, S.T., & Gray, C. (2023). Applications for Colon Organoid Models in Cancer Research. Organoids, 2(1):37-49. doi: 10.3390/organoids2010003. https://www.mdpi.com/2674-1172/2/1/3
- McCauley, H.A., Riedman, A.M., Enriquez, J.R., Zhang, X., Watanabe-Chailland, M., Sanchez, J.G., Kechele, D.O., Paul, E.F., Riley, K., Burger, C., Lang, R.A., & Wells J.M. (2023). Enteroendocrine Cells Protect the Stem Cell Niche by Regulating Crypt Metabolism in Response to Nutrients. Cellular and Molecular Gastroenterology and Hepatology, 15(6):1293-1310. doi: 10.1016/j.jcmgh.2022.12.016. https://pubmed.ncbi.nlm.nih.gov/36608902/
- Mukhare, R., Gandhi, K.A., Kadam, A., Raja, A., Singh, A., Madhav, M., Chaubal, R., Pandey, S., & Gupta, S. (2025). Integration of Organoids With CRISPR Screens: A Narrative Review. Biology of the Cell, 117(4):e70006. doi: 10.1111/boc.70006. https://pmc.ncbi.nlm.nih.gov/articles/PMC11995251/
- Sprangers, J., Zaalberg, I.C., & Maurice, M.M. (2021). Organoid-based modeling of intestinal development, regeneration, and repair. Cell Death & Differentiation, 28:95–107. doi: 10.1038/s41418-020-00665-z. https://www.nature.com/articles/s41418-020-00665-z
- Zheng, L., Zhan, Y., Wang, C., Fan, Q., Sun, D., Li, Y., & Xiong, Y. (2024). Technological advances and challenges in constructing complex gut organoid systems, Frontiers in Cell and Developmental Biology, 12. doi: 10.3389/fcell.2024.1432744. https://www.frontiersin.org/journals/cell-and-developmental-biology/articles/10.3389/fcell.2024.1432744/full