What are mitochondria?
The mitochondria, also commonly known as “the powerhouse of the cell”, is a compartment within a cell known as an organelle.
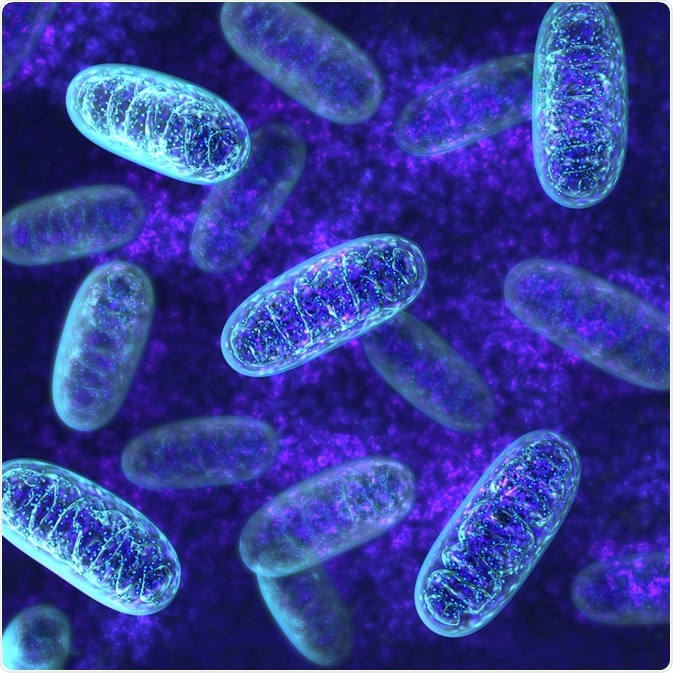
Image Credit: 3d_man/Shutterstock.com
Mitochondria are responsible for turning sugars, fats, and proteins into different forms of chemical energy, including cellular energy adenosine triphosphate (ATP). Each eukaryotic cell contains different numbers of mitochondria.
Apart from generating cellular energy, mitochondria are also involved in a type of programmed cell death called apoptosis.
Stimuli such as loss of growth factors and chemicals required for cell survival or damage by radiation and free radicals can cause changes in the inner mitochondrial membrane.
This leads to the opening of the mitochondrial permeability transition (MPT) pore and downstream pro-apoptotic protein activation.
Where do they come from?
One of the most accepted theory is that mitochondria are once free-living organisms that were engulfed by eukaryotes and developed an endosymbiotic relationship with the host cells, meaning they coexist in a mutually beneficial manner.
The mitochondria provide chemical energy to the host and the host provides food and protection. The main evidence that supports this hypothesis is that mitochondria have their DNA and divide independently of the host cell.
Interaction with the nuclear genome
The mitochondrial and nuclear genome interact in many ways such as genetic transfer, gene expression regulation, and encoding composite proteins together, meaning part of the protein is encoded in the mitochondrion and part in the nucleus.
Cytochrome oxidase is an example that contains contain both mitochondrial- and nuclear-encoded subunits.
The mitochondria require nuclear-encoded genes to function
Mitochondrial genes can be divided into three categories - ribosomal RNA (rRNA), transfer RNA (tRNA) and protein-coding genes. Mitochondrial DNA (mtDNA) encodes 3 to 67 protein genes and 0-27 tRNA genes.
The mitochondrial genomes also contain 10 to 1000-fold fewer genes than their proposed ancestor alphaproteobacteria.
The loss of genes and the diverse number of genes different mitochondria encode suggest the use of nuclear-encoded proteins that might have been mtDNA transferred into the nuclear genome.
In all characterized mitochondrial genomes, the two major rRNA genes encoding the small and large subunit of the ribosome are found.
Nevertheless, the amount of tRNA encoded by mtDNA varies and some mitochondria do not encode any tRNA genes, which are essential for protein synthesis.
Although there is no evidence of tRNA genes being transferred into the nuclear genome, it is accepted that the mitochondria use nuclear-encoded tRNA for mitochondrial protein synthesis.
Moreover, in yeast mitochondrial proteome, mitochondrial ribosomal proteins, aminoacyl-tRNA synthetases, and respiratory Krebs cycle components are nuclear-encoded.
The mechanism of which nuclear-encoded protein functions in the mitochondria is proposed to be duplication of a nuclear gene that one copy of the gene functions in the cytoplasm while another copy acquired mitochondrial targeting sequence, which allows it to translocate into the mitochondria and carry out its functions.
Another proposed mechanism is that a single gene uses alternative promoters or post-expression alterations to produce two protein versions of the same gene, supplying the same protein to both the cytoplasm and the mitochondria.
Transfer of mitochondrial genes to the nucleus
During evolution with eukaryotic cells, many mitochondrial genes have been transferred into the host genome.
This can result in two ways: the transferred gene being functional or becoming a non-functional pseudogene.
MtDNA can escape the mitochondria during disruptions of the mitochondrial membranes, an occasional fusion of mitochondrial and nuclear membrane, or during cellular stress that damages the mitochondrial membrane.
When inside the nucleus, pieces of mtDNA can integrate into the genome via DNA repair mechanisms.
If the piece of mtDNA is integrated into a genome location containing gene expression regulatory elements such as the promoter, the mtDNA can be expressed.
Transfer of nuclear genes to mitochondria
There is only one report of a gene in the mitochondrial gene that may have been transferred from the nucleus: the mutS gene in corals, which encodes a mismatched DNA repair protein.
Other than this exception, the transfer of genes between mitochondria and the nucleus is unidirectional. One reason to account for this may be because mtDNA escapes to the nucleus via vacuole release but there is no comparable process for the nucleus.
Also, nuclear genes contain non-coding sequences called introns and they need to be spliced out when a gene is expressed into messenger RNA (mRNA) for the protein to be functional. There is no splicing machinery in the mitochondria.
Regulation of gene expression
The mitochondrion can regulate nuclear gene expression via metabolites.
It is found that the level of mitochondrial metabolite tRNA leucine correlates with histone posttranslational modifications, thus affecting how histones organize nuclear DNA structure and influencing gene expression.
Moreover, mitochondrial oxidants are known to be key activators of JNK and ERK1/2 pathways, which lead to downstream apoptosis or oxidant detoxification pathways.
In addition, in a study done in fruit flies, it is found that nuclear genes in which expression changes according to mtDNA variation are clustered on certain chromosome arms.
These clusters and flanked by non-coding RNA, suggesting a role of mtDNA in regulating non-coding genes.
On the other hand, signaling from the nucleus can also affect mitochondrion gene expression.
Cellular energy levels denoted by adenosine diphosphate (ADP) to ATP and NAD+/ NADH ratios are known to regulate AMPK and sirtuin pathways, which work together to regulate a process which cells increase mitochondrial mass called mitochondrial biogenesis.
Implication
Mitochondrial replacement therapies are promising to treat mtDNA-associated diseases in humans. However, unfavorable interactions between mtDNA and the nuclear genome pose a challenge.
It is essential to understand the interaction between the mitochondria and the nucleus for the development of these therapies.
References:
- Adams, K. L. & Palmer, J. D. (2003) Evolution of mitochondrial gene content: gene loss and transfer to the nucleus. Plant Molecular Evolution. Available from: http://www.sciencedirect.com/science/article/pii/S1055790303001945.
- Dyall, S. D., J, Brown MT FAU - Johnson, Patricia & Johnson, P. J. Ancient invasions: from endosymbionts to organelles.
- Elmore S. (2007). Apoptosis: a review of programmed cell death. Toxicologic pathology, 35(4), 495–516. https://doi.org/10.1080/01926230701320337
- Fetterman, J. L. & Ballinger, S. W. (2019) Mitochondrial genetics regulate nuclear gene expression through metabolites. Proceedings of the National Academy of Sciences. 116 (32), 15763-15765. Available from: http://www.pnas.org/content/116/32/15763.abstract. Available from: DOI: 10.1073/pnas.1909996116.
- Mossman, J. A., Biancani, L. M. & Rand, D. M. (2019) Mitochondrial genomic variation drives differential nuclear gene expression in discrete regions of Drosophila gene and protein interaction networks. BMC Genomics. 20 (1), 691.
- MRC Mitochondrial Biology Unit. What are Mitochondria?. Available from: http://www.mrc-mbu.cam.ac.uk/what-are-mitochondria#collapseOne [Accessed 24-2-2020].
Further Reading