Life-threatening explosives contaminate millions of hectares around the world and biological agents are increasingly used to address such issues. Plants can be used as bioremediatory agents to process harmful explosive materials as well as sentinels for the detection and monitoring of explosive materials.
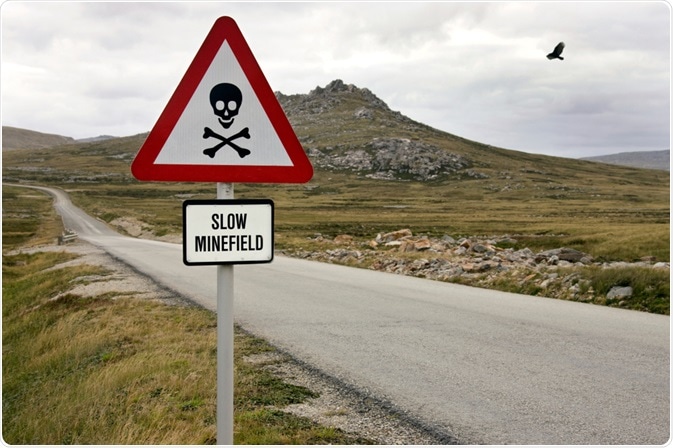
Minefield. Image Credit: Steve Allen/Shutterstock.com
Explosives and bioremediation
Each year, an estimated 15,000 to 20,000 people are injured or killed from discarded landmines around the world. Currently, approximately 10 million hectares of military land in North America is contaminated with munition components. Such threats are not just recent, but munitions disposed of in World War 1 at Verdun still threaten human health in the surrounding area to this day.
Explosive-contaminated sites and their associated environmental problems are further described in a 2012 book by R. Albright. The author details current remediation methods used to reduce the extent of explosives pollution. Common methods include landfill, incineration, and advanced oxidation, but the effectiveness of such strategies can be context-dependent.
Scientists have developed a range of bioremediation strategies to address explosives. These rely on biological organisms to detect, quantify, and process pollutants including explosive materials.
Plants are particularly useful as they can be used as sentinels to detect what lies beneath the surface thanks to root systems. Indeed, roots uptake water, nutrients, and chemicals from the subsurface soil and therefore act as detectors of the surrounding area. To date, researchers have focused on a range of approaches including the identification of genes, biochemical detoxification pathways, and field-applicable plant species to make key species agents of bioremediation.
Harnessing plant responses to detect explosives
In plants, compounds that can cross root membranes can elevate organismal stress by altering characteristics including physiological and morphological traits. Known examples of such alterations include reductions in chlorophyll content, decreased stomatal conductance, increase in fluorescence, and overall reductions in biomass.
Explosives can enter plants by crossing root membranes via water transport and are then stored in roots or translocated to leaves, where they then accumulate. One explosive that is able to translocate readily is Royal Demolition Explosive (RDX), known chemically as cyclotrimethylenetrinitramine, and is a common explosive in the military.
RDX is one of the first versions of plastic explosives and forms the basis of many explosive materials and is commonly mixed with TNT and other materials. RDX is known to cause plant stress at relatively low concentrations and moves into plants and is translocated into aerial portions of plants where it negatively affects leaf function and health.
Once the uptake of RDX by plants is complete, it is reduced to MNX (hexahydro-1-nitroso-3,5-dinitro-1,3,5-triazine) and dinitroso (DNX), where it is then mineralized into formaldehyde, methanol, and carbon dioxide. These elements can then be released by plants through the soil, transforming a potentially dangerous material.
Further studies have also elaborated on this process by describing the role of endophytic and rhizospheric microorganisms in the detoxification of explosives as well as the genetic engineering of relevant plant species that can be deployed in the field. Together, these strategies can be used to mitigate the extent of pollution from common explosives.
This bioremediation process generates plant stress, which can be detected through the use of sensors such as hyperspectral sensors. Such tools can identify pollutants on varying spatial scales and are ideal for remotely testing large spaces and has been adapted for field surveys. However, certain elements may affect the accuracy of such assessments.
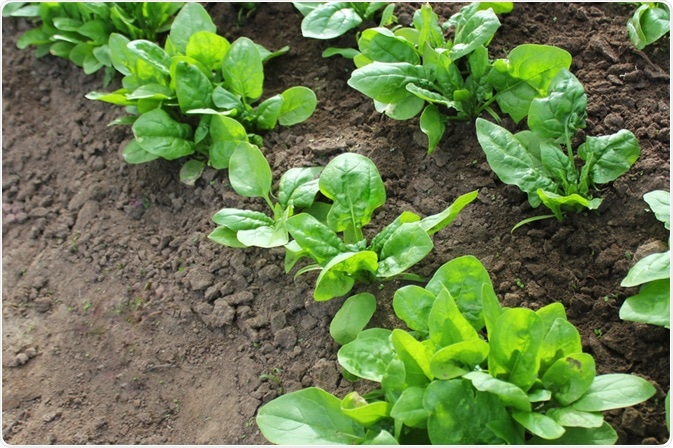
Spinach Plant. Image Credit: vaivirga/Shutterstock.com
Factors of complexity and variation in the detection of explosives
To be effective in detecting a specific contaminant remotely, methods such as plant monitoring possess some inherent sources of variation.
For example, it is important to distinguish between contamination sites and potential also positives or negatives. As plant responses can be species- and explosive-specific, discriminating between natural, i.e., drought or nutrient deficiency, and anthropogenic stressors is crucial for the accurate remote detection of explosives.
Moreover, interindividual differences may also vary across species and regions. A report of 18 herbaceous dicotyledonous plants across North America found that growth was inhibited in 16 of the 18 species at RDX levels upwards of 100 mg/kg. Although the majority of species responded similarly, the rate and severity of effects differed widely.
Such variations may be due to life-history traits of species, which require consideration when monitoring stress levels in response to explosives contamination. Indeed, angiosperms were found to accumulate RDX in aerial tissues, which differed from coniferous trees that had RDX localized primarily in the root systems.
Further differences are also found in the bioremediation process itself as the initial reductive steps are light-independent, suggesting the involvement of plant biological activities.
The future of explosive bioremediation
Recent studies have focused on two main approaches in progressing plant bioremediation. That is, improving methods of detection for field-based strategies, and the genetic engineering of species to transform explosive contaminants more effectively.
In a 2019 study, American researchers were able to employ whole-plant hyperspectral imaging for the first time to sense changes in mays when exposed to explosives. In response to exposure to 250 mg/kg of RDX explosive contamination, plants experienced a decrease in Green-Red Vegetation Index (GRVI), Photochemical Reflectance Index (PRI), Modified Red Edge Simple Ratio (MRESR), and Vogelmann Red Edge Index 1 (VREI1). Such findings are particularly useful for developing explosive-specific indices to accurately identify plants in contaminated soil using aerial-based hyperspectral systems.
The second approach relies on the enhancement of phytodetoxification processes in plants through genetic modification. Key pathways have already been identified in Arabidopsis and Poplar that are involved in the classic detoxification mechanism, including cytochrome P450s, reductases, peroxidases, and GSTs. However, the exact enzymes potentially able to transform RDX have yet to be isolated.
Emerging issues are also limiting the progress of bioremediation processes and remain to be addressed. For instance, the accumulation of contaminants in the ecosystem poses environmental issues as stored RDX becomes biologically available through the food chain through herbivory or is returned, like RDX, back to the soil when the plant dies.
In a world where global environmental pollution has reached unprecedented levels, bioremediation strategies are increasingly beneficial, not only for explosive materials but other pollutants as well. Technical advancements and the refinement of detection and bioremediation methods will further bolster the range and quality of applicable strategies.
Sources:
- Albright, R. (2011). Cleanup of Chemical and Explosive Munitions: Location, Identification and Environmental Remediation (2nd ed.).
- William, A. Halasz, A., Manno, D., et al. (2012). Biodegradation of RDX Nitroso Products MNX and TNX by Cytochrome P450 XplA. Environmental Science & Technology, 46(13), 7245–7251. doi:10.1021/es3011964
- Leydon, A. R., Gala, H. P., Guiziou, S., & Nemhauser, J. L. (2020). Engineering Synthetic Signaling in Plants. Annual Review of Plant Biology, 71(1), 767–788. doi:10.1146/annurev-arplant-081519-035852
- Manley, P. V., Sagan, V., Fritschi, F. B., & Burken, J. G. (2019). Remote Sensing of Explosives-Induced Stress in Plants: Hyperspectral Imaging Analysis for Remote Detection of Unexploded Threats. Remote Sensing, 11(15), 1827. doi:10.3390/rs11151827
- Rylott, E. L., & Bruce, N. C. (2019). Right on target: using plants and microbes to remediate explosives. International Journal of Phytoremediation, 21(11), 1051–1064. doi:10.1080/15226514.2019.1606783
Further Reading