By Dr. Said QabbaahReviewed by Lexie Corner
Cryo-electron microscopy (cryo-EM) is a high-resolution imaging method that captures biological macromolecules in a near-native, hydrated state.
Unlike X-ray crystallography or NMR spectroscopy, cryo-EM involves rapidly freezing samples and imaging them at cryogenic temperatures, eliminating the need for crystallization and preserving structural integrity.1,2
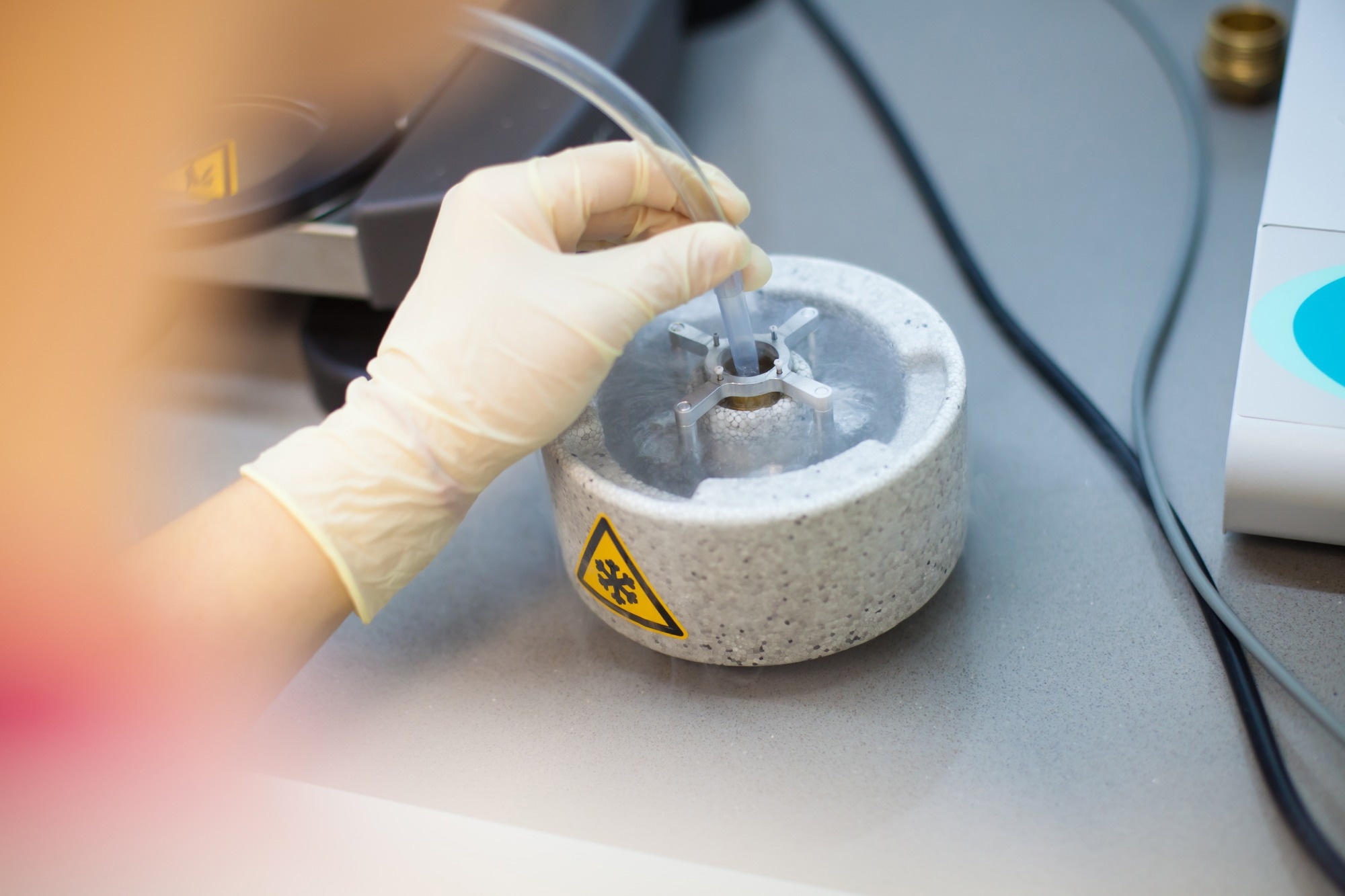
Image Credi: PolakPhoto/Shutterstock.com
In structural biology, cryo-EM has become a key tool for examining the three-dimensional architecture of viruses.2 It is particularly suited to large, symmetric particles and enables detailed analysis of capsids, envelope proteins, and complexes involved in viral entry and replication.3
These insights have been instrumental in guiding vaccine development.
Understanding Viral Structure with Cryo-EM
Cryo-EM enables near-atomic resolution imaging of viruses, often reaching 3–4 Å, where individual amino acid side chains and secondary structural elements, such as α-helices and β-sheets, become visible.
Through single particle analysis (SPA), thousands to millions of two-dimensional projections of viral particles in random orientations are computationally aligned and combined to generate high-resolution three-dimensional density maps. These reconstructions support atomic modeling of capsid proteins and help identify key residues involved in assembly and morphogenesis.
This approach is particularly effective for symmetric viruses, although ongoing advances in image processing now allow for reliable reconstructions of more heterogeneous structures.
Cryo-electron tomography (cryo-ET) complements SPA by capturing pleomorphic viruses and viral replication complexes directly within cells. This technique preserves the native spatial organization of proteins, lipids, and nucleic acids.
Sub-tomogram averaging further refines these 3D reconstructions, resolving features such as multimeric protein complexes at membrane necks and filamentous viral RNA templates.
These capabilities allow for direct visualization of conformational changes, protein–protein and protein–RNA interactions, and dynamic events such as membrane fusion and genome packaging, offering detailed insights into viral replication and pathogenesis.3,4
See how cryo-EM is used in real-world virology research:
Virology Using Cryo-EM at the Institute of Science and Technology Austria
Structural Insights into Medically Relevant Viruses
Cryo-EM has significantly advanced the structural characterization of several clinically important viruses, uncovering mechanistic features that were previously difficult to resolve and directly informing therapeutic and vaccine development.
In HIV research, cryo-EM has provided high-resolution views of the envelope glycoprotein in its native conformation.
These insights have supported the design of vaccine candidates (including mRNA-based platforms) and informed the development of entry-targeted therapies such as fostemsavir (Rukobia).5,6 Cryo-EM has also enabled structure-based antigen design by identifying conserved regions critical for neutralizing antibody responses.
For influenza, structural studies using cryo-EM have resolved the conformation of hemagglutinin, the surface glycoprotein responsible for host cell entry. This has improved understanding of antigenic drift and informed the development of next-generation vaccines, such as nanoparticle-based constructs that target conserved epitopes for broader and longer-lasting immunity.7
In the case of coronaviruses, particularly SARS-CoV-2, cryo-EM was pivotal in mapping the spike protein and its interaction with the human ACE2 receptor. This structural information guided stabilization of the spike in its prefusion conformation, a key design feature of several effective COVID-19 vaccines, including Comirnaty.8,9
Cryo-EM also enabled the identification of spike protein regions that elicit neutralizing antibodies, supporting structure-based antigen design and targeted immunogen engineering. In parallel, it contributed to the development of antibody-based therapies, such as casirivimab–imdevimab (Ronapreve/REGEN-COV), by clarifying antibody binding sites and mechanisms of viral neutralization.10,11
Download your PDF copy now!
What Are the Current Limitations of Cryo-EM?
While cryo-EM has reshaped structural biology, several technical and practical challenges still limit its broader use.
One significant challenge is sample preparation. Achieving optimal vitreous ice thickness, uniform particle distribution, and minimal aggregation is technically demanding. Even minor inconsistencies can introduce artefacts or obscure high-resolution features. The preparation process itself is time-intensive and typically requires expert handling.1,2,12
Another limitation is its resolution, particularly for smaller macromolecules. Cryo-EM is generally less effective at resolving low-mass proteins compared to X-ray crystallography. Beam-induced motion and radiation damage can further reduce image quality, although motion correction algorithms and low-dose imaging techniques have helped address this.1,2,12
Data processing also poses a barrier. High-end computing infrastructure is needed for particle alignment, classification, and 3D reconstruction. This creates an accessibility gap for smaller labs or institutions with limited resources. Moreover, interpreting cryo-EM density maps—particularly for flexible or heterogeneous molecules—often requires complementary structural or biochemical data.13
Finally, cost remains a major limitation. Cryo-EM instrumentation and maintenance are expensive, and although shared facilities help, demand often outpaces capacity. This can lead to long wait times and limited throughput for new projects.13
Love This Kind of Content? Subscribe to One of Our Expert-Curated Newsletters
Emerging Technologies and Developments
Recent innovations in cryo-EM have addressed several technical challenges and broadened its utility across structural biology and virology.
The introduction of direct electron detectors has significantly improved image quality. By increasing sensitivity and signal-to-noise ratios, these detectors enable high-resolution imaging at lower electron doses, helping to mitigate radiation damage and preserve structural detail.12
Advances in image processing algorithms now allow more accurate 3D reconstructions from a wider variety of samples, including those that are structurally heterogeneous or present in low abundance.12 AI and machine learning tools are increasingly used to automate particle picking, classification, and model refinement, streamlining data analysis and accelerating research workflows.14
The development of advanced sample preparation techniques, such as cryo-focused ion beam milling and improved vitrification methods. This has enhanced the preservation of biological specimens in their native states, facilitating the study of complex structures and enabling the analysis of larger and more diverse biological systems.12
Together, these developments continue to expand the resolution and versatility of cryo-EM. In virology, they support more detailed views of viral architecture and enable structure-guided design of vaccines and therapeutics with greater precision.
Want to see how cryo-EM is driving scientific discovery across biology? Don’t miss these cutting-edge developments:
References and Further Reading
- Chari, A., & Stark, H. (2023). Prospects and Limitations of High-Resolution Single-Particle Cryo-Electron Microscopy. Annual Review of Biophysics, 52:391-411. doi: 10.1146/annurev-biophys-111622-091300
- Benjin, X., & Ling, L. (2019). Developments, applications, and prospects of cryo-electron microscopy. Protein Science, 29(4):872-882. doi: 10.1002/pro.3805
- Dutta, M., & Acharya, P. (2024). Cryo-electron microscopy in the study of virus entry and infection. Frontiers in Molecular Biosciences, 11. doi: 10.3389/fmolb.2024.1429180
- Zhu, D., Cao, D., & Zhang, X. (2023). Virus structures revealed by advanced cryoelectron microscopy methods. Structure, 31(11):1348-1359. doi: 10.1016/j.str.2023.09.008
- Islam, F., Das, S., Ashaduzzam, M., Sillman, B., Yeapuri, P., Nayan, M.U., Oupický, D., Gendelman, H.E., & Kevadiya, B.D. (2024). Development of an extended action fostemsavir lipid nanoparticle. Communications Biology, 7, 917. doi: 10.1038/s42003-024-06589-5
- Highland, C.M., Tan, A., Ricaña, C.L., Briggs, J.A.G., & Dick, R.A. (2023). Structural insights into HIV-1 polyanion-dependent capsid lattice formation revealed by single particle cryo-EM. Proceedings of the National Academy of Sciences of the United States of America, 120(18):e2220545120, doi: 10.1073/pnas.2220545120
- Wu, N.C., & Wilson, I.A. (2020). Structural Biology of Influenza Hemagglutinin: An Amaranthine Adventure. Viruses, 12(9):1053; doi: 10.3390/v12091053
- Bodakuntla, S., Kuhn, C.C., Biertümpfel, C., & Mizuno, N. (2023). Cryo-electron microscopy in the fight against COVID-19-mechanism of virus entry. Frontiers in Molecular Biosciences, 10:1252529. doi: 10.3389/fmolb.2023.1252529
- Ponnampalli, S., Birudukota, N.V.S., & Kamal, A. (2022). COVID-19: Vaccines and therapeutics. Bioorganic & Medicinal Chemistry Letters, 75:128987, doi: 10.1016/j.bmcl.2022.128987
- Li, N., Li, Z., Fu, Y., & Cao, S. (2020). Cryo-EM Studies of Virus-Antibody Immune Complexes. Virologica Sinica, 35:1-13. doi: 10.1007/s12250-019-00190-5
- Antanasijevic, A., Bowman, C.A., Kirchdoerfer, R.N., Cottrell, C.A., Ozorowski, G., Upadhyay, A.A., Cirelli, K.M., Carnathan, D.G., Enemuo, C.A., Sewall, L.M., Nogal, B., Zhao, F., Groschel, B., Schief, W.R., Sok, D., Silvestri, G., Crotty, S., Bosinger, S.E., & Ward A.B. (2022). From structure to sequence: Antibody discovery using cryoEM. Science Advances, 8(3). doi: 10.1126/sciadv.abk2039
- Guaita, M., Watters, S.C., & Loerch, S. (2022). Recent advances and current trends in cryo-electron microscopy. Current Opinion in Structural Biology, 77:102484. doi: 10.1016/j.sbi.2022.102484
- Meng, X., Ratnayake, I., Escobar Galvis, M.L., Kotecki, J., Ramjan, Z., & Zhao, G. (2023). Best practice: setting up and operating a mid-sized cryo-EM facility. Frontiers in Molecular Biosciences, 10:1302680. doi: 10.3389/fmolb.2023.1302680
- Chung, J.M., Durie, C.L., & Lee, J. (2022). Artificial Intelligence in Cryo-Electron Microscopy. Life, 12(8):1267. doi: 10.3390/life12081267