AZoLifeSciences speaks to Dr. David J. Rawlings from the Seattle Children’s Research Institute about recent developments in engineered T cells for type 1 diabetes.
What led you to begin this research?
For the past 20 years, the goal of the research in my lab has been to develop cures for childhood diseases of the immune system. We have approached this by studying in parallel the mechanistic basis for immune diseases, and how to develop genetic therapies that could correct defective immune cells.
We began developing gene-editing methods in 2005 and expanded this work as part of an NIH Roadmap initiative in 2010.
Initially, gene editing to insert designer DNA into specific sites of the human genome was very inefficient, making it not clinically viable. In 2015, however, our lab reported a method for gene editing using co-delivery of designer nucleases and a viral-encoded donor DNA.
Our new approach greatly improved the editing efficiency of primary T cells and hematopoietic stem cells. Based on extensive previous work by others, we knew that the transcription factor, FOXP3, was essential to program cells with a regulatory T cell phenotype.
Thus, we predicted that a potential future application of our gene-editing technology might be to engineer stable regulatory T cells to treat autoimmune diseases. Coincidentally, we had an existing Type 1 diabetes research collaboration with Jane Buckner’s lab at the Benaroya Research Institute at Virginia Mason (BRI), which led to our current collaboration on this project.
Gene Editing at Seattle Children's
Why would it be so revolutionary if children with type 1 diabetes could be treated using T cells rather than insulin injections?
Type 1 diabetes (T1D) is an autoimmune disease that destroys the insulin-producing cells in the pancreas. The primary treatment for T1D is to provide lifelong daily insulin treatments to patients to replace this missing hormone. It is a considerable burden of care on patients and parents, who effectively never get a rest from the demands of staying healthy and safe.
Our goal is to take a patient’s T cells, modify them, and then return the engineered cells to the patient where they will help protect insulin-producing cells from immune injury. That advancement, if performed at an early stage during the disease development, would free people with T1D from having to take insulin to manage blood sugar and improve their quality of life.
What is the role of T cells in Type I Diabetes?
In T1D, specific types of immune cells called effector T cells mistakenly attack insulin-producing islet cells in the pancreas. The job of these islet cells is to sense when glucose levels are rising in the bloodstream and to respond by releasing insulin.
Once destroyed by the unchecked effector T cells, the islet cells are no longer present to release insulin. Glucose levels in the bloodstream then rise unabated, causing the symptoms of diabetes such as frequent urination, unquenched thirst, insatiable hunger, and extreme fatigue.
A healthy immune system has a back-up mechanism for protecting tissues that are under attack from self-reactive effector T cells: regulatory T cells. Regulatory T cells inhibit the inflammatory responses of effector T cells, effectively halting the tissue destruction.
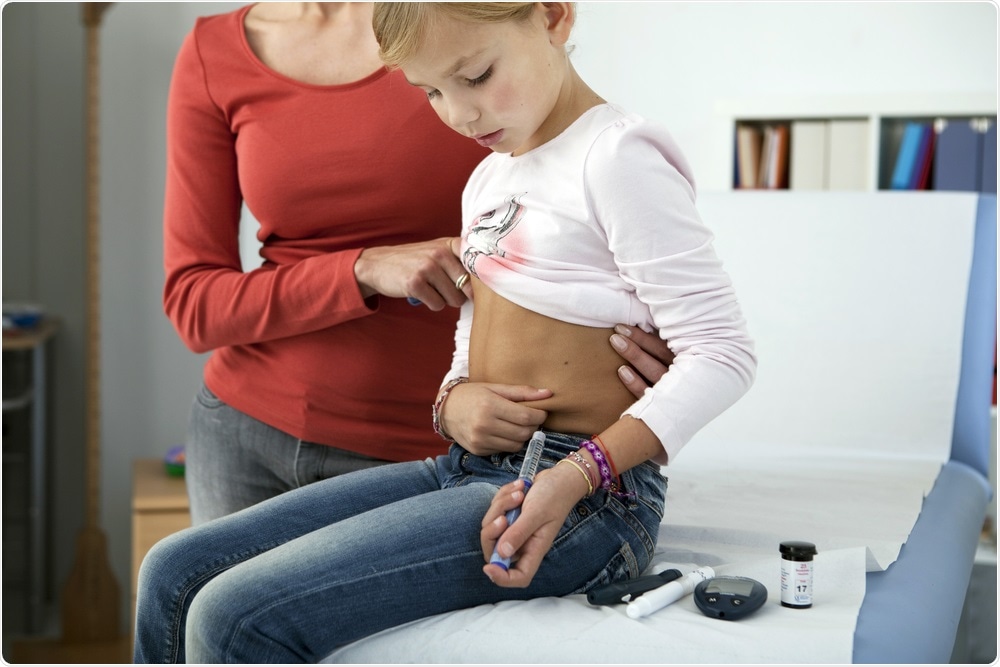
Image Credit: Image Point Fr/Shutterstock.com
How does genetic engineering of T cells work as a therapeutic strategy?
Genetically engineered T cells to fight disease have already shown great promise against childhood leukemia. Instead of generating engineered chimeric antigen receptor (CAR) T cells to stimulate the immune system to seek and destroy cancer cells in a child with leukemia, treating autoimmune conditions like T1D will require us to reprogram a patient’s T cells to suppress the overactive immune response.
What is the FOXP3 gene and how did you use it to carry out this strategy?
The FOXP3 gene produces a protein that is known as the “master regulator” of regulatory T cell development and function. Patients with mutations in FOXP3 that prevent the gene from making this protein develop severe inflammatory disease, including T1D, very early in life.
Interestingly, conventional T cells are prevented from stably expressing the FOXP3 protein by “epigenetic silencing”, which effectively means that the gene is not accessible to the cellular transcriptional machinery used to turn a gene on.
We used genetic engineering to insert a piece of DNA (a transcriptional promoter) into the FOXP3 gene of conventional T cells that allowed the transcriptional machinery to access and turn on the FOXP3 gene. This had the effect of forcing FOXP3 protein to be made in the conventional T cells and caused the cells to look and behave like normal regulatory T cells.
The hope is that when transferred back into the patient, these engineered or edited regulatory-like T cells (edTreg) enter the pancreas, where they can help to suppress the overactive immune response, sustaining and protecting the function of the islet cells.
What is the antigen-specific feature of your method and why is this important?
T cells have a unique protein on their cell surface, called a T cell receptor (TCR), which recognizes foreign proteins (or antigens) and instructs the cell to begin an immune response. Each T cell makes only one of thousands of possible TCRs.
In autoimmune diseases such as T1D, effector T cells express TCRs that recognize proteins made naturally by pancreatic islet cells. Regulatory T cells with TCRs that also recognize antigens on pancreatic islet cells will traffic to the pancreas. Additionally, when the TCR of a regulatory T cell is activated by its antigen, it is instructed to start suppressing immune responses.
Thus, having the right TCR on the edTreg will provide the strongest immune suppression to the target tissue. The best T-cell receptor will direct the edTreg to the pancreas and turn on their protective activity and, ideally, will also work for the greatest number of T1D patients.
.jpg)
Image Credit: ratlos/Shutterstock.com
How has your research been tested so far?
Our recent paper published in Science Translational Medicine demonstrated that edTreg generated using our FOXP3 gene-editing approach looked similar to natural Treg. They also functioned like natural Treg in both animal models and tissue cultures.
In an animal model of graft-versus-host-disease, transplantation of effector T cells leads to severe inflammation by cells that recognize the animal’s tissues as foreign. Delivery of edTreg prevented these symptoms and maintained survival.
We also showed how we could make the cells antigen-specific in an animal model of multiple sclerosis. In this model, the antigen-specific edTreg decreased the activity of the disease-causing T cells more so than the non-specific edTreg.
Data presented at the 2020 American Society for Gene and Cell Therapy Annual Meeting (abstract 905) tested antigen-specific edTreg in an animal model of T1D. The islet-specific T cells homed to the pancreas and persisted, blocking diabetes triggered by islet-specific effector T cells while nonspecific edTreg and naturally occurring Treg failed to do so. This research also showed how we could translate this approach to generate islet-specific human edTreg.
How far off is your research from reaching clinical trials?
We’re optimistic that we can complete the preclinical and safety studies and clinical trial design required to submit an Investigational New Drug Application to the U.S. Food and Drug Administration over the next three years.
This will allow us to seek approval to open a phase 1 clinical trial using this new approach.
How could your research improve treatment for and the lives of Type I Diabetes patients in the long term?
We envision that this therapy would be used to preserve the pancreatic islets of new patients that are developing T1D. Patients would have their blood drawn, and then later receive an infusion of their own T cells, genetically engineered to suppress the immune cells driving T1D.
We hope that this would establish a long-lasting immunological “tolerance” to the islet cells. Instead of the life-long requirement for daily insulin therapy, the T cell therapy has the potential last for years or, potentially, be curative.
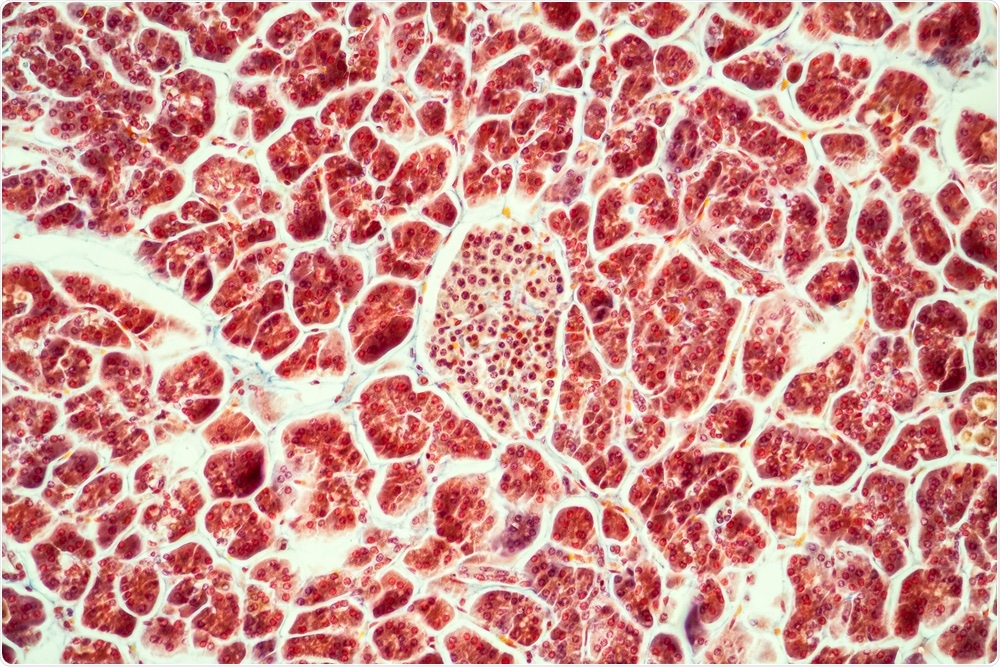
Image Credit: Dr. Norbert Lange/Shutterstock.com
What are the next steps for your research?
In the next phase of research, teams from Seattle Children’s and BRI will work to fine-tune the T-cell receptor used for the edTreg and enhance the manufacturing process used to generate the edTreg for clinical use. These studies will help establish the final cell product and key information required to establish a first-in-patient clinical trial.
In the longer-term, we hope to develop methods to create universal “off the shelf” Treg therapies, where one T cell product could be used for multiple patients, which would improve patient accessibility and reduce the costs of this therapy.
Where can readers find more information?
https://pulse.seattlechildrens.org/engineered-t-cells-for-type-1-diabetes-move-closer-to-clinic/
https://www.seattlechildrens.org/research/centers-programs/immunity-and-immunotherapies/
About Dr. David J. Rawlings
David J. Rawlings, MD, is chief in the Division of Immunology, overseeing the immunodeficiency clinic at Seattle Children’s Hospital. He is also director of the Center for Immunity and Immunotherapies leading the immunology research programs at Seattle Children’s Research Institute.
Dr. Rawlings is professor of pediatrics and adjunct professor in the Department of Immunology at the University of Washington School of Medicine.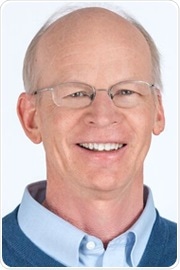
He earned his MD with honors from the University of North Carolina School of Medicine and completed a residency and chief residency in pediatrics at the University of California, San Francisco. He was an intramural research fellow at the NIH and a senior fellow at the Howard Hughes Medical Institute, UCLA.
He completed specialty training in pediatric rheumatology and immunology at Children’s Hospital Los Angeles and directed the pediatric rheumatology program at UCLA. Dr. Rawlings has been the recipient of many awards including election to the American Society for Clinical Investigation, Association of American Physicians, and the Children’s Hospital Guild Association Endowed Chair in Pediatric Immunology Research.
Dr. Rawlings also co-directs the Northwest Genome Engineering Consortium, a research program funded as part of the NIH Roadmap for Medical Research and focused on developing enzymatic reagents and delivery methods for site-specific gene repair in hematopoietic stem cells. His publications include more than 90 peer-reviewed papers and many invited reviews.
His primary research interests include dysregulated B cell development and signaling leading to immunodeficiency, autoimmunity or lymphoid malignancies, and the development of gene therapy for primary immune deficiency diseases.
His laboratory uses expertise in basic and clinical immunology, signal transduction and lymphocyte developmental biology to understand how altered signals can lead to immunologic disease, with the ultimate goal of developing translational therapies capable of specifically modulating these disorders.
He is also actively involved in collaborative studies with the bone marrow transplant team to design and test new protocols for stem cell transplant in non-malignant disorders.
Dr. Rawlings is a member of multiple regional and national organizations, an NIH study section member, and an ad hoc reviewer for various grant programs and immunology journals.