In this interview, we speak to Professor Jason Micklefield about his latest research that may be able to produce new antibiotics through gene-editing technologies.
Please can you introduce yourself and tell us what inspired your latest research into antibiotics and gene editing?
I am a Professor of Chemical Biology at the University of Manchester. I started my career as an organic chemist developing methods to synthesize complex molecules called natural products which have inspired the development of many important therapeutic agents. I realized that synthesis was laborious, inefficient, and often involved dangerous or toxic chemical reagents.
In light of this, I chose instead to explore how nature assembles natural products using enzymes in biosynthetic pathways. During these studies, I became interested in a class of enzymes called non-ribosomal peptides synthetases (NRPS) which produce many highly complex peptide products that have important biological activity. Many NRPS products have been used to develop pharmaceuticals such as antibiotics, anticancer and immunosuppressive agents.
Antimicrobial resistance is described by the World Health Organization (WHO) as “one of the biggest threats to global health, food security, and development today.” What is meant by the term ‘antimicrobial resistance’ and are there any factors that accelerate the rate of resistance?
Antimicrobial resistance occurs when bacterial, fungal, and other microbial pathogens become resistant to antimicrobial drugs, such as antibiotics. Antimicrobial resistance is a natural phenomenon, which can be accelerated through the overuse or misuse of antimicrobial drugs.
For example, antibiotics are often prescribed for viral infections (cold and flu) which have been mistaken for bacterial infections. Antibiotics are also widely used in agriculture, in animal feed for instance, which can lead to the evolution of resistance in pathogens that infect animals, which can eventually transfer to human pathogens.
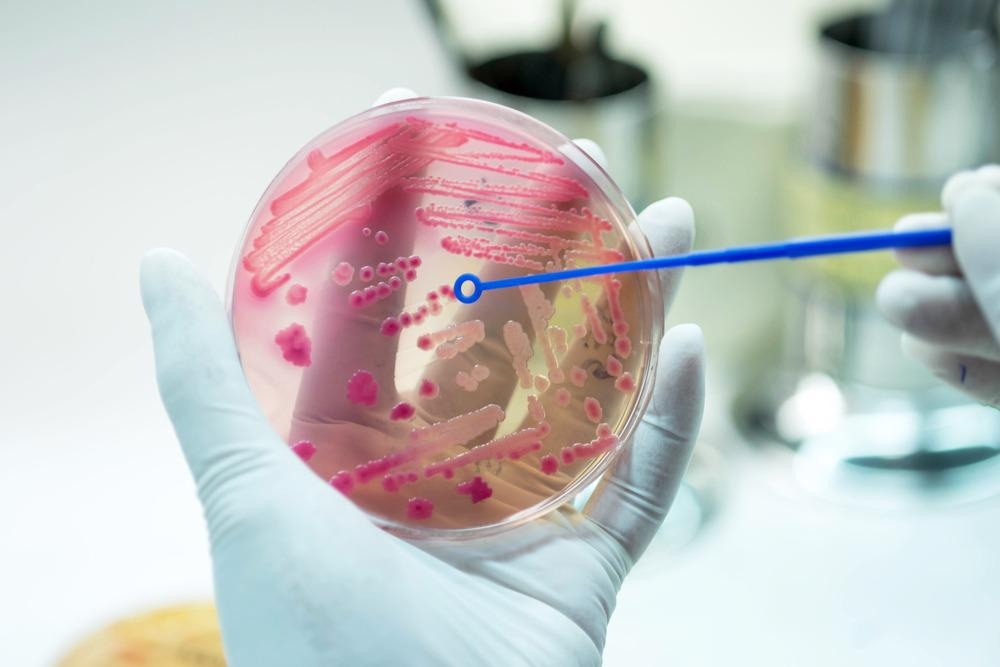
Image Credit: AnaLysiSStudiO/Shutterstock.com
Why are new medicines urgently required to help tackle antimicrobial resistance? What benefits would new antibiotics have for global health?
Improving how antibiotics are prescribed (stewardship) so that they are only used when required, and reducing the use of antibiotics in animals can help slow the development of antimicrobial resistance. However, for life-threatening infectious diseases where current antibiotics fail, there is no option but to develop new and more effective antibiotic treatments.
If we had new classes of antibiotics in reserve, then we might be able to combat emerging deadly drug-resistant pathogens. Unfortunately, the development of new antibiotics is expensive and as these medicines are normally administered over a short time course, there is little commercial incentive to drive future research and development efforts.
What are nonribosomal peptide synthetase (NRPS) enzymes and what role do they play within antibiotic drug discovery?
Nonribosomal peptide synthetases (NRPS) are large and highly complex enzymes that assemble standard and unusual amino acids substrates into highly complex peptide products, including many of the most important antibiotics in the clinic today (e.g. penicillin, vancomycin, and daptomycin). NRPS are comprised of a series of modules organized into an assembly line architecture, with each module introducing a single amino acid precursor into a growing peptide chain.
The modular organization suggests that manipulation of these NRPS could be relatively straightforward. In principle, the replacement of modules within the NRPS assembly line should enable a different amino acid building block to be introduced at a selected position, leading to user-defined peptide products.
For this reason, many research groups have sought to develop methods for engineering (re-programming) NRPS to make new and improved antibiotics for example.
Up until now, manipulating the structures of NRPS enzymes has proven challenging. Why is this, and how has your new research addressed this problem?
Unfortunately, manipulating NRPS enzymes has proved challenging. In most cases, the engineered NRPS variants have completely abolished activity or greatly reduced product yields. Moreover, most previous NRPS engineering efforts have focused on relatively small NRPS enzymes (comprised of only a few modules) which are manipulated and produced in simple host species (e.g. E. coli).
However, the majority of the most therapeutically important nonribosomal peptides are produced by non-model organisms, particularly Streptomyces bacteria. The genomes of Streptomyces species are GC-rich and the NRPS genes are also highly repetitive in sequence, making genetic manipulation via traditional methods highly challenging.
We aimed to manipulate NRPS encoding genes directly in the native Streptomyces producer strain, as the native producer contains all of the unusual amino acids and other “ingredients” as well as the regulatory components necessary for production.
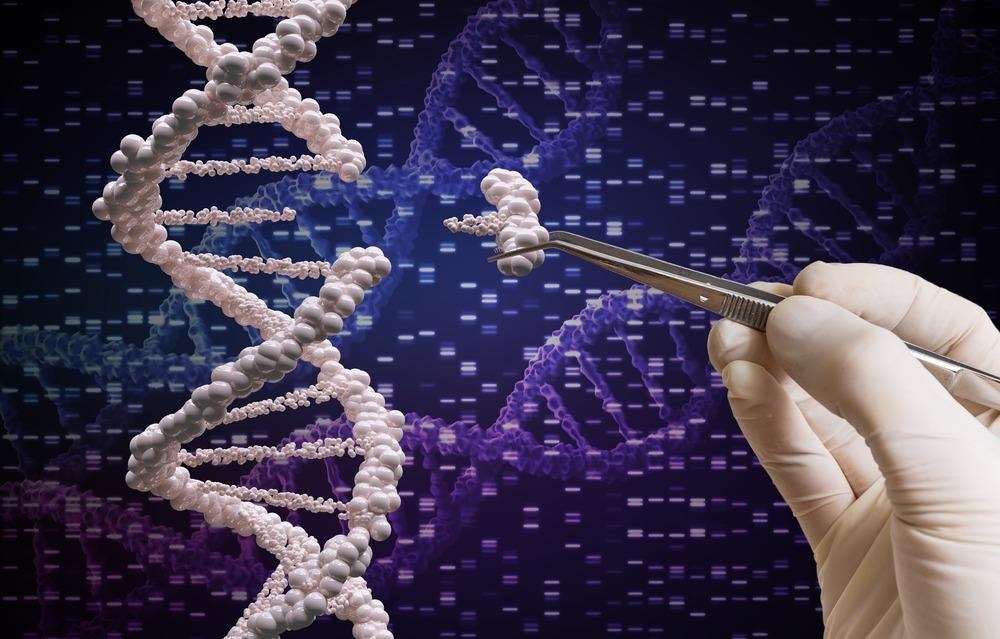
Image Credit: vchal/Shutterstock.com
Can you describe how you carried out your latest research into gene editing and antibiotics? What did you discover?
Our idea was to use CRISPR-Cas9 to edit the genes encoding for NRPS assembly lines in vivo, as this would enable precise replacement of key parts of the NRPS enzyme much more efficiently than using traditional methods.
The increase in editing efficiency allowed us to manipulate the very large NRPS encoding genes involved in the biosynthesis of enduracidin in Streptomyces fungicidicus. Enduracidin is a lipopeptide antibiotic that is produced by an enormous NRPS consisting of 17 modules that assemble 17 amino acid precursors. Enduracidin is similar in structure and bioactivity to ramoplanin, which entered phase 3 clinical trials, and so it could be a potential lead-structure in developing new antibiotic treatments.
By swapping out a small region within each module, a “subdomain” that recognizes the amino acid substrates, we created new NRPS enzymes that produced modified peptide products with one amino acid building block swapped for another. We found that the new CRISPR-Cas9 strategy was much more efficient and successful in delivering new lipopeptide antibiotics than the more traditional engineering approaches that had been used previously. Overall, we were able to produce 10 new lipopeptide variants by swapping subdomains.
CRISPR-Cas9 technology has become more prominent within the medical and life sciences industry since its discovery in 2012. How do you feel CRISPR has changed the scientific industry and how do you see its role changing over the next year 10 years?
We have found CRISPR-Cas9 to be a fast and reliable way to engineer biosynthetic pathways including NRPS and other enzymes in Streptomyces bacteria, which are prolific antibiotic producers. Overall CRISPR is easier and much more rapid to implement than the more traditional genetic approaches used to manipulate bacterial genomes.
We have been fortunate that CRISPR works well in the Streptomyces strains we have explored so far. However, there are some strains where this method has been less successful. Despite this, new and improved CRISPR systems are been developed, so there is no doubt this will be the method of choice moving forward. The ease with which CRISPR-Cas9 can be used will mean that more and more labs will try to use it in the future.
For example, previously only experts in microbial genetics were able to engineer complex antibiotic assembly lines in challenging bacterial strains such as Streptomyces species. However, as the technology becomes easier to use, teams who are not experts in genetics, like us, can use it too.
How could your research help in the development of a new generation of antibiotic treatments?
During our work, we discovered that the sequence similarity of the NRPS subdomains being swapped was very important. Those subdomain swaps with higher similarity (i.e. those which were most closely related to the enduracidin subdomains) were much more likely to function within the engineered NRPS and produce new antibiotics. This information should be a useful guide for future efforts using gene editing to engineer NRPS and possibly related assembly line enzymes such as polyketide synthases (PKS).
Previously, researchers may have felt that engineering large NRPS might be too difficult and high risk. However, our work can provide confidence and help guide the engineering of a vast range of NRPS enzymes and also other enzymes such as PKS that are common in antibiotic biosynthetic pathways. In light of this, we think it should be possible, even for those who are not genetics experts, to use our approach and deliver new antibiotics structures that can be further developed for possible clinical use.
Do you believe that your research will help to further our understanding of antimicrobial resistance leading to further options to help tackle it whilst also helping to achieve the Sustainable Development Goals (SDGs)?
Although our work does not address how antimicrobial resistance evolves per se, it could lead to the provision of new antibiotics which are essential in combating the problem. Our work aligns well with the sustainable development goals (SDGs). Firstly, many antibiotics are produced using multistep chemical synthesis, which is expensive, relies on deleterious reagents and solvents that are increasingly unsustainable.
Our approach can deliver new antibiotics, in a single-step fermentation process (aqueous media), which is environmentally benign, requires less energy, and does not use deleterious reagents or create polluting waste. If high yielding fermentation processes can be established, then the antibiotics can be produced at a large scale and low costs, and this should make new treatments more widely available for poorer sections of the population (e.g. the Global South) where there is the greatest need.
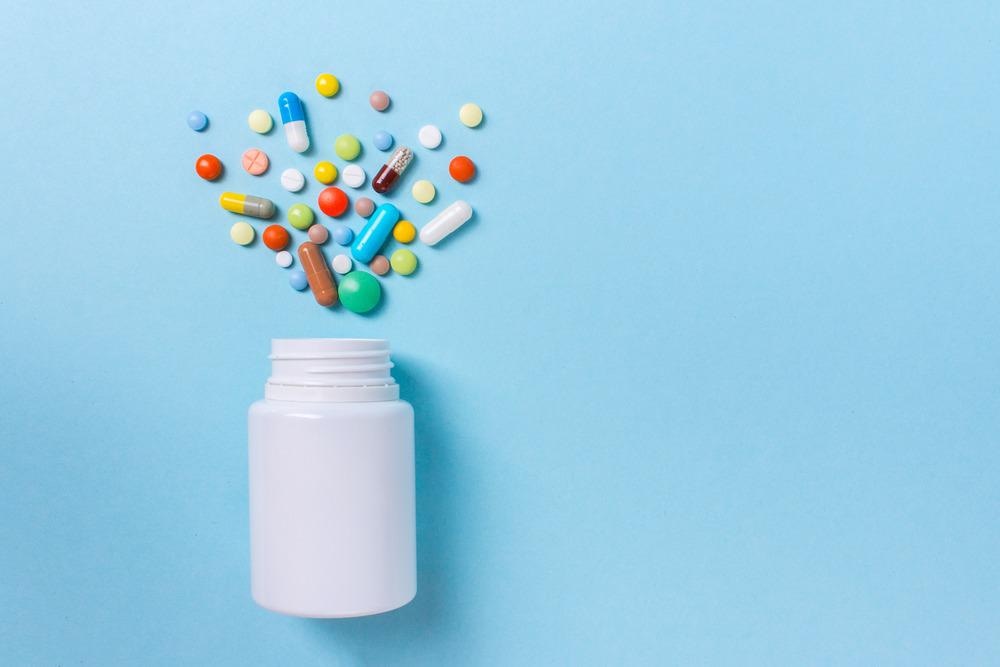
Image Credit: ADragan/Shutterstock.com
What are the next steps for you and your research into antibiotics?
We have identified a number of other important antibiotic target compounds that are potentially useful in treating fungal and parasitic infections which lead to diseases that are particularly problematic in the developing world.
For example, we are working on producing safer, less toxic variants of antifungal agents that are used to treat mucormycosis, also known as black fungus, which was associated with the death of many COVID-19 patients, particularly in India. In addition, we are also exploring ways of engineering improved antiparasitic agents for the treatment of Leishmaniasis, which is a parasitic disease that is very problematic in tropical regions of the Global South.
Where can readers find more information?
About Professor Jason Micklefield
Jason graduated from the University of Cambridge in 1993 with a Ph.D. in Chemistry, working with Prof Sir Alan R. Battersby FRS to complete the first total synthesis of haem d1, a co-enzyme molecule essential for the survival of bacterial species. He won a NATO postdoctoral fellowship to investigate biosynthetic pathways and enzyme mechanisms at the University of Washington, USA, with Prof Heinz G. Floss..jpg)
In 1995 he began his independent research career as a Lecturer in Organic Chemistry at Birkbeck College, University of London, before moving to Manchester in 1998. He was promoted to Senior Lecturer (2002), Reader (2003), and then to Professor of Chemical Biology in 2008. He was recipient of the Royal Society of Chemistry (RSC) Bader Award (2019) for “innovative contributions to the elucidation of natural product biosynthetic pathways & development of enzymes for synthesis”; the RSC Natural Products Report Lecture Award (2008) for “significant contribution to natural products research.” He was also appointed Visiting Professor at East China University of Science and Technology (ECUST), Shanghai, China (2018-present).