It is estimated that, in the United States alone, 108 billion pounds of food are wasted each year, equating to 408 billion dollars. With agriculture, forestry, and general land use encompassing the second largest cause of greenhouse gas emissions, we must seek a greater integration in green chemistry within the fields of food science. This will be done through the education of consumers, the improvement of food storage, and the implementation of green biofuels within the industry.
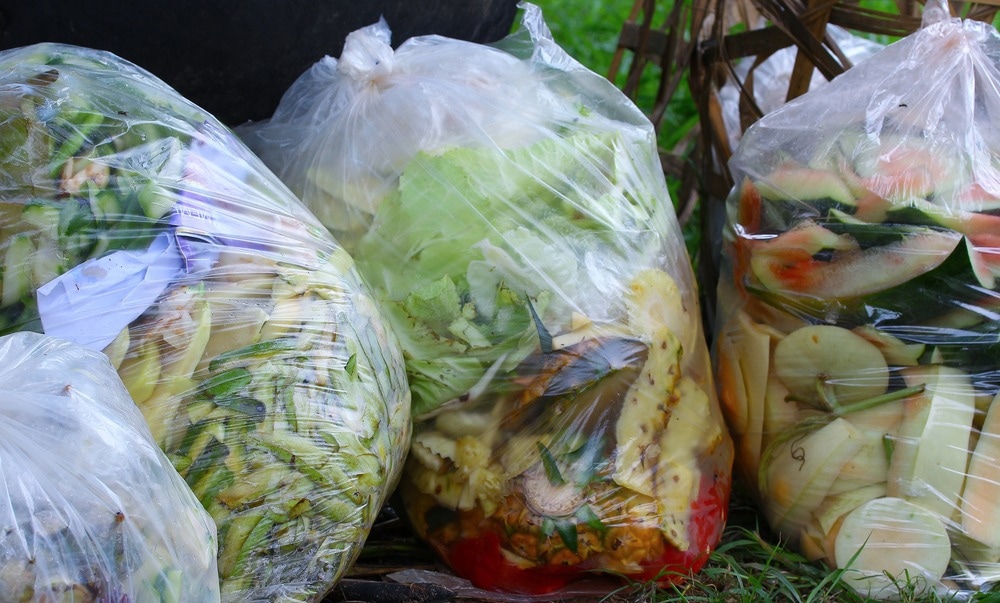
Image Credit: Boonchuay1970/Shutterstock.com
The Problem with Depositing Food Waste
A wasted piece of fruit or peel produced by a food supply chain (FSC)s will most likely end up in a landfill, adversely affecting the soil quality or polluting the surrounding water. This will result in a release of methane gas as the waste decomposes. This waste would take a different form if we consumed the food ourselves, contributing to the mineralization process in the nitrogen cycle by converting organic material into usable nitrogen-containing molecules. Not only is the process of depositing food waste into landfills hostile to the earth’s health, it is also non-sustainable.
To tackle these issues head-on, Paul Anastas and John Warner formulated twelve principles of green chemistry. They are the following:
- Prevention
- Atom Economy
- Less Hazardous Chemical Syntheses
- Designing Safer Chemicals
- Safer Solvents and Auxiliaries
- Design for Energy Efficiency
- Use of Renewable Feedstocks
- Reduce Derivatives
- Catalysis
- Design for Degradation
- Real-time analysis for Pollution Prevention
- Inherently Safer Chemistry for Accident Prevention
How to Make Use of Food Supply Chains
One feasible way to make further use of FSCs (abiding by principles three, six, and seven) is to convert the possible waste into fuels and fertilizers. This has been done using anaerobic digestion, changing the heterogeneous biomass through a microbial process. This process provides a healthy alternative to the long-established fossil fuel industry.
To make this process possible, waste must be collected and processed on a much larger scale. This is so high-value chemical compounds can be synthesized at a high volume to justify costs. Examples of biomass in which this technique could be implemented are agricultural waste produced by large farms, citrus peels from mass juicers, or spent grain amongst breweries.
Other recycling approaches that are becoming more commonplace is waste straw being used to replace wood products for boards and furniture. Boards bound together using waste biomass ash are much more favorable than toxic silicate binders.
The chemicals that make up orange peels, such as limonene, pectin, and bioethanol, can undergo green secondary processes, the byproducts of which can be used for cleaning products, detergents, and personal care products. Bioethanol, one of the more versatile biofuels, can be used as motor fuel as an additive to gasoline, while pectin can be used in the pharmaceutical industry in the form of emulsions, cosmetics, or capsules.
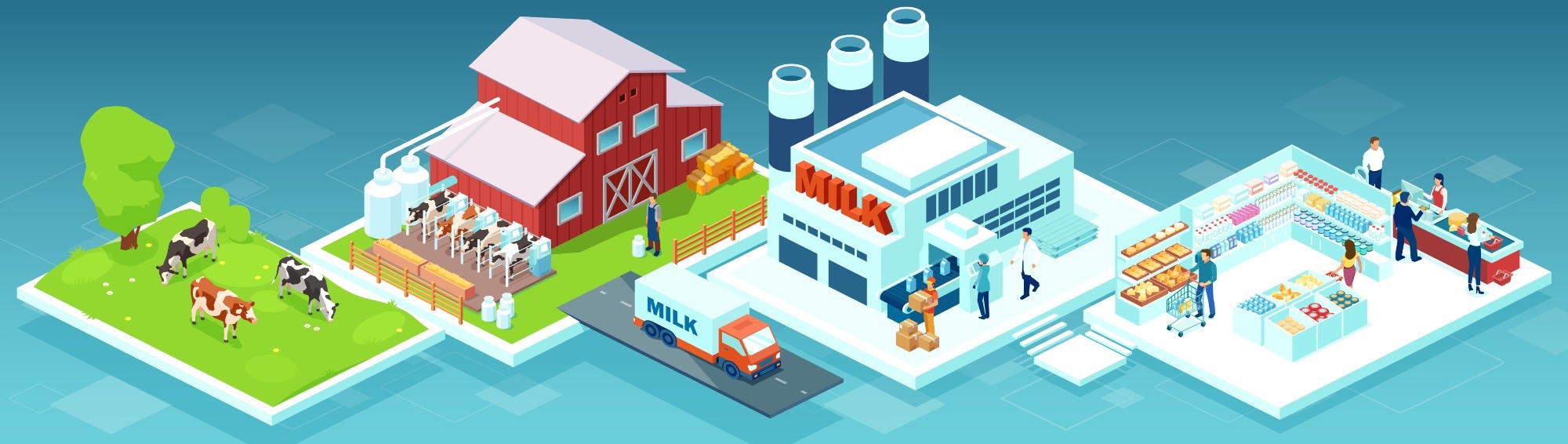
Image Credit: FGC/Shutterstock.com
Greener Methods of Acid Production
Founded upon data by the Department of Food Science and Biotechnology in Korea, the Catalysis Engineering and Chemical Engineering Department of Delft University of Technology, and the Center for Green Chemistry, vast industry improvements can be made by utilizing non-hazardous acids. Energy costs and production prices can be cut by using organic acids at low temperatures (Malik, Citric) rather than mineral acids (hydrochloric acid, sulfuric acid) at higher temperatures. Pectin, for example, has shown yields with higher molecular weight and viscosity, contributing to high efficiency when used in green chemistry processes. Tasking FSC fuels as feedstocks for chemical reactions may be the future in the fuel and energy sectors of the world.
Colorimetric Sensors in Fruit Waste
Green chemists have been constructing ways to combat this dilemma at home for those not directly aligned with the food production industry. Climacteric changes can occur in fruit like bananas, apples, kiwi, and melon, indicating how ripe it is. Though ripening is easy to determine in some fruits, it is much harder in others. The ripening and souring process in a fruit’s lifecycle correlates to the amount of ethylene gas produced. Ethylene is a crucial plant hormone, present in all steps within the developmental process. These levels determine when fruit should be harvested, stored, and disposed of. If we knew when to throw fruit away, when to eat it, and when to store it, subsequent waste could be drastically diminished.
Viewing this from a molecular scope, ethylene receptors are found on the endoplasmic reticulum (ER), and certain genes will be transcribed in the presence of ethylene. Without ethylene, these receptors will cause EIN2 integral proteins to remain on the ER. Therefore the F-box proteins in the nucleus cause transcription factors to be degraded. When ethylene is present, it will go into the receptor, triggering a phosphorylation response that cleaves the EIN2 protein from the ER. As a consequence, transcription factors can activate the ethylene response gene. This is an autocatalytic cycle, where the product (ethylene) is also the catalyst.
Though gas chromatography, electrochemical methodologies, and photoacoustic spectroscopy can all be used to monitor this process, they require funds and chemical knowledge that the average consumer does not possess. For these reasons, simple colorimetric sensors have been developed to immediately portray color change that indicates ethylene levels.
The methods explored in this paper are just a fraction of what is being assayed in the broader scope of green chemistry and food sciences, and it could be argued that much of the science regarding these fields is still within its infancy. However, the more funding and research allocated to these fields, the closer we become to a sustainable, green planet.
Sources:
- Andrea Álvarez, Atul Bansode, Atsushi Urakawa, Anastasiya V. Bavykina, Tim A. Wezendonk, Michiel Makkee, Jorge Gascon, and Freek Kapteijn Chemical Reviews (2017). Challenges in the Greener Production of Formates/Formic Acid, Methanol, and DME by Heterogeneously Catalyzed CO2 Hydrogenation Processes 117 (14), 9804-9838 DOI: 10.1021/acs.chemrev.6b00816
- Anastas, P. T. and Warner, J. C. Green Chemistry: Theory and Practice. Oxford University Press: New York, 1998, p. 30. By permission of Oxford University Press.
- Carpick, R; Darryl, S; Marcus, M; Ericksson, M.A. Polydiacetylene films (2004): A review of recent investigations into chromogenic transitions and nanomechanical properties. Journal of Physics: Condensed Matter. 16
- Nguyen, L.H; Oveissi, F; Chandrawati, R; Dehghani, F; Naficy, S. (2020) Naked-Eye Detection fo Ethylene Using Thiol-Functionalized Polydiacetylene-Based Flexible Sensors. ACS Sensors., 5, 7, 1921-2928.
- Park, I; Park, H. J; Jeong W.; Nam, Jihye. Low Temperature Thermochromic Polydiacetylenes: Dsign, Colormetric Properties, and Nanofiber Formation. Macromolecules. 2016, 49, 4, 1270-1278
- Huang, Q; Wu, W.;Ai, L; Liu J. Highly Sensitive Polydiacetlyene Ensembles for Biosensing and Bioimaging. Front. Chem. 2020.
- Nguyen, L.H; Oveissi, F; Chandrawati, R; Dehghani, F; Naficy, S. (2020) Naked-Eye Detection fo Ethylene Using Thiol-Functionalized Polydiacetylene-Based Flexible Sensors. ACS Sensors., 5, 7, 1921-2928.
- Matharu AS, de Melo EM, Houghton JA. Opportunity for high value-added chemicals from food supply chain wastes. Bioresour Technol. 2016 Sep;215:123-130. doi: 10.1016/j.biortech.2016.03.039. Epub 2016 Mar 12. PMID: 26996261.
- Andiappan, V., How, B. S., & Ngan, S. L. (2021). A Perspective on Post-Pandemic Biomass Supply Chains: Opportunities and Challenges for the New Norm. Process Integration and Optimization for Sustainability, 5(4), 1003–1010. https://doi.org/10.1007/s41660-021-00176-5
Further Reading