Genome-editing technology has generated new opportunities to engineer disease resistance traits in crops and solve major challenges for crop improvement to meet the ever-increasing global food demand.
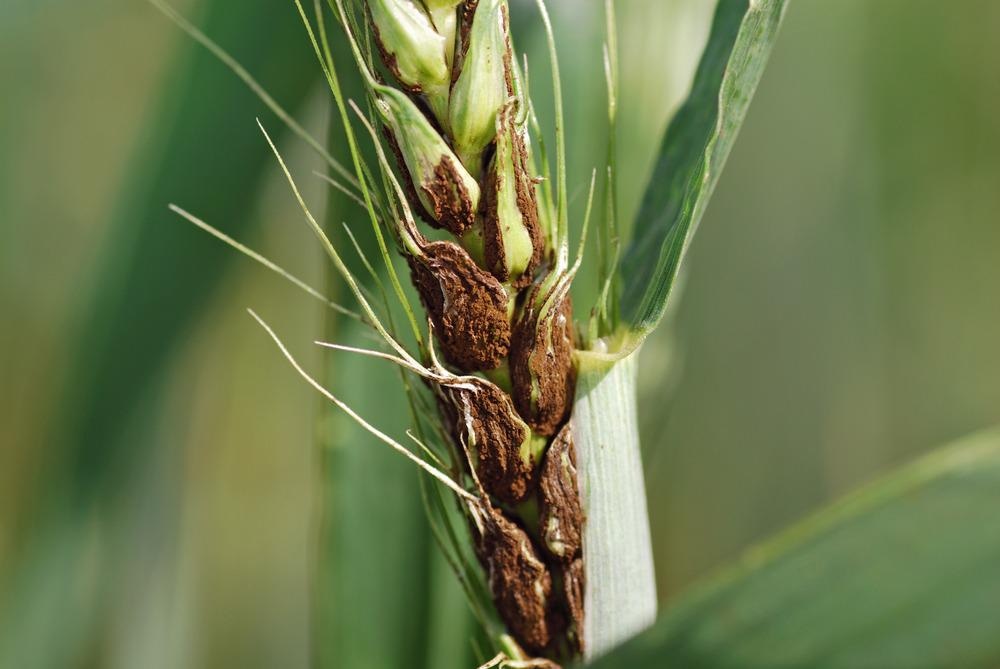
Image Credit: Damian Herde/Shutterstock.com
The emergence of new breeding techniques to enhance crop resistance
Food production around the world is increasingly reliant upon a limited number of crops, which are grown abundantly to cater for a growing human population. In addition to the rise of monocultures, food production is threatened by climate change, which is expected to increase the diversity of crops and pathogens, as well as increase the frequency and severity of extreme weather events.
These factors are expected to increase the likelihood of food insecurity. In response, food scientists and crop breeders are focusing on applying genomic tools to improve the resilience of plants as well as increase the quality and quantity of crops.
Since the rise of domestication, plant breeding has been the most successful approach for developing new crop varieties, which in turn, allows for major advances in societal development and progression.
Nonetheless, crops are susceptible to a large set of pathogens including fungi, bacteria, and viruses, which cause important economic losses. The enhancement of plant resistance to these pathogens, therefore, plays an important role in adjusting crop production to meet global population increases.
However, many plant pathogens have the ability to evolve through mutation or recombination resulting in resistances to the plant defenses or the phytosanitary product used in food production. When this occurs, disease control measures become ineffective as genotypes evolve increasingly quickly through natural selection and are able to spread to other locations, causing large-scale crop losses.
Traditional breeding programs prior to integrating molecular tools focused on the identification of natural and induced mutant alleles for resistance, which were then selected for continuous thorough breeding techniques. These approaches were time-consuming and imprecise, leading for instance, to incidents including the transfer of large genome regions instead of just single gene insertions that then affect plant development.
With the emergence of genomic techniques for plant breeding programs, a number of new tools became available. Specifically, association genetics employing single nucleotide polymorphisms (SNPs) and other molecular markers became increasingly used for plant breeding, creating high throughput data fundamental for the identification of quantitative trait loci (QTL).
From SNPs and the identification of QTLs, an array of new breeding techniques (NBTs) was developed which allowed for breakthroughs in disciplines including such as developmental biology, abiotic stress tolerance, or plant-pathogen resistance.
In a 2018 review by Virginia Borelli et al., researchers detailed the mechanisms and the range of applications of NBTs. The authors describe how NBTs employ site-directed nucleases to introduce double-stranded breaks at predetermined sites in DNA. Host cells then repair the breaks resulting in small insertions or deletions via near homologous end-joining (NHEJ) or micro-homology-mediated end-joining (MMEJ), or a modified gene carrying predetermined nucleotide changes copied from a repair matrix via homologous recombination (HR).
Further developments then emerged to increase the number and accuracy of changes including meganucleases (MNs), zinc finger nucleases (ZFNs), transcription activator-like effector nucleases (TALENs) and clustered regularly interspaced short palindrome repeats (CRISPR)/CRISPR-associated protein 9 (Cas9), which make up the four types of nucleases used in genome editing.
Since its emergence, CRISPR-Cas9 technology has increased in accuracy, applicability, popularity, and has now become the most widespread tool for gene editing. The low financial costs and high success rate in gene modification has led to CRISPR/Cas9 editing becoming a powerful tool for future enhancement of agronomic traits in crops.
Current applications and examples of crop resistance using molecular techniques
Since the integration of CRISPR-encoding sequences that introduce targeted mutations in the host plant genome, a wide range of disease-resistant crops and plants have been developed.
To effectively test and implement genetic modifications for disease resistance, researchers have categorized resistances according to the type of threat. The most common are bacterial diseases, fungal pathogens, and plant viral diseases. Each category was extensively reviewed by Yin and Qiu in 2019, who presented the current status of diseases resistance measures and the examples of resistances across threat types.
Bacterial diseases present the most challenging resistance as they are difficult to control after the establishment of epidemics since bacterial pathogens are highly diverse, multiply rapidly, and can be spread in many ways. This makes resistances difficult to develop and researchers have focused instead on the molecular mechanisms of host–bacterial pathogen interactions to indirectly limit the impacts of bacterial pathogens.
Recent studies on tackling bacterial pathogens include the improvement of resistances to citrus canker, which is a devastating disease caused by the bacterium Xanthomonas citri ssp. citri (Xcc). Researchers have modified the CsLOB1 gene, which is a member of the lateral organ boundaries domain (LBD) family of transcription factors, providing resistance to Xcc.
Fungal pathogens also present a unique challenge. They are the most frequent causal agents of plant diseases due to diverse lifestyles and high genetic flexibility, which allows them to rapidly invade new hosts, break gene-mediated resistances and generate resistance to fungicides, thus constituting a major challenge in disease control.
Of particular value has been the development of resistance to powdery mildew, which is a global fungal disease, with studies focusing on the identification and introduction of resistance genes from alien species into resistant strains of a crop by hybridization. However, since most of these resistance genes are strain-specific, resistances gradually decline as new varieties of wheat powdery mildew evolve in the field, emphasizing the need for alternative approaches.
Finally, plant viral diseases are the last category of interest in disease resistance studies. Viruses evolve most rapidly of all diseases and insect vectors are often involved as disease vectors, making viral spread even faster and more effective. To counter these effects, transgenic expression of virus proteins or RNAs has been used to improve plant virus resistance, and the resultant resistance is called pathogen-derived resistance. More recently though, studies have used RNA interference (RNAi) induced by double-stranded RNA as an efficient method to confer resistance against viruses to plants and provides a new tool in the arsenal against viral pathogens.
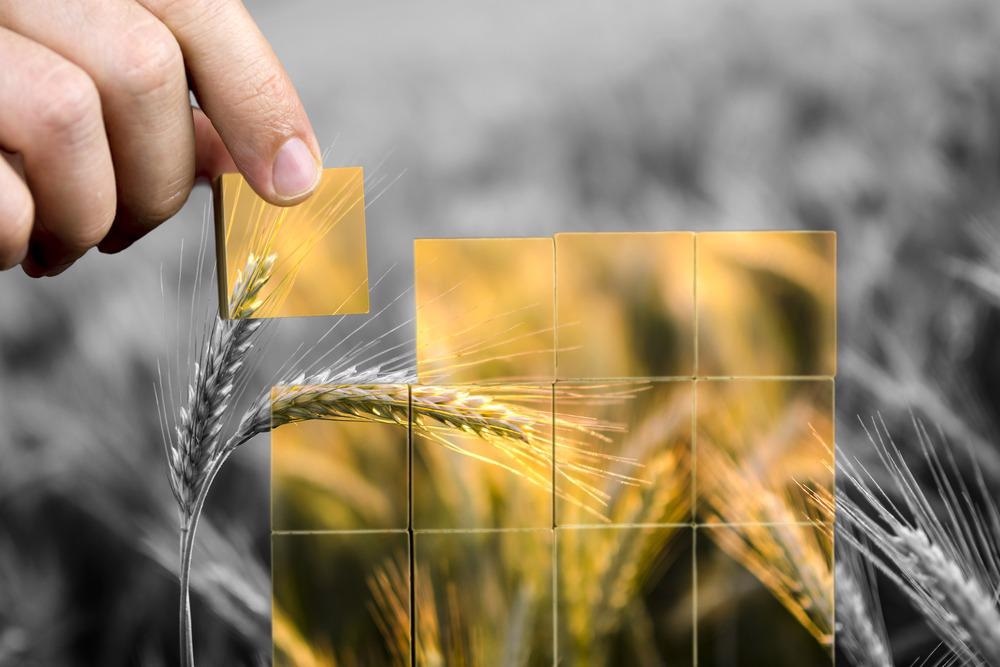
Image Credit: Gajus/Shutterstock.com
Implications of genetic modifications for crop resistance into the future
Genome editing provides unique opportunities and potential to overcome the limitations of conventional disease-resistance breeding and limit the threat of plant disease to food security, which are expected to increase because of climate change. However, some challenges remain.
Currently, disease-resistant crops against non-viral pathogens are generated via genome editing through targeted mutagenesis of genes that negatively regulate defense, referred to as S genes. One of the main challenges facing genome editing for resistance is many target genes including S genes are often involved in plant growth and development, and unfavorable or null mutations in S genes may cause adverse effects on plant growth.
This hinders the application of gene editing for resistance, which has become a central paradigm of plant pathology. To overcome this challenge, researchers have now begun considering more complex interactions involved in genome editing to counter plant diseases.
Specifically, diseases rely on a virulent pathogen, a susceptible host, and a favorable environment. Genome editing can therefore be applied to constrain any of the three factors or several together, resulting in the interruption of plant-pathogen interactions to control diseases.
Considering greater levels of complexity and overcoming existing barriers in diseases resistance will allow bio-edited disease-resistant crops to become a standard tool in plant breeding. Thus, genome editing has the potential to limit the severity of plant diseases and ensure more stable food security in the future.
Sources:
- Borrelli, V. M. G., Brambilla, V., Rogowsky, P., Marocco, A., & Lanubile, A. (2018). The Enhancement of Plant Disease Resistance Using CRISPR/Cas9 Technology. Frontiers in Plant Science, 9. https://doi.org/10.3389/fpls.2018.01245
- Langner, T., Kamoun, S., & Belhaj, K. (2018). CRISPR Crops: Plant Genome Editing Toward Disease Resistance. Annual Review of Phytopathology, 56(1), 479–512. https://doi.org/10.1146/annurev-phyto-080417-050158
- Yin, K., & Qiu, J. L. (2019). Genome editing for plant disease resistance: applications and perspectives. Philosophical Transactions of the Royal Society B: Biological Sciences, 374(1767), 20180322. https://doi.org/10.1098/rstb.2018.0322
- Zaidi, S. S. E. A., Mahas, A., Vanderschuren, H., & Mahfouz, M. M. (2020). Engineering crops of the future: CRISPR approaches to develop climate-resilient and disease-resistant plants. Genome Biology, 21(1). https://doi.org/10.1186/s13059-020-02204-y
Further Reading