Analytical chemistry is the branch of chemistry that studies the composition and structure of matter. Through a series of advanced instruments and methods, it enables the identification and quantification of substances.
This field is crucial to a wide range of sectors, from healthcare to environmental science and food safety. From detecting contaminants in drinking water to analyzing drug composition, analytical chemistry plays a vital role in solving real-world problems.
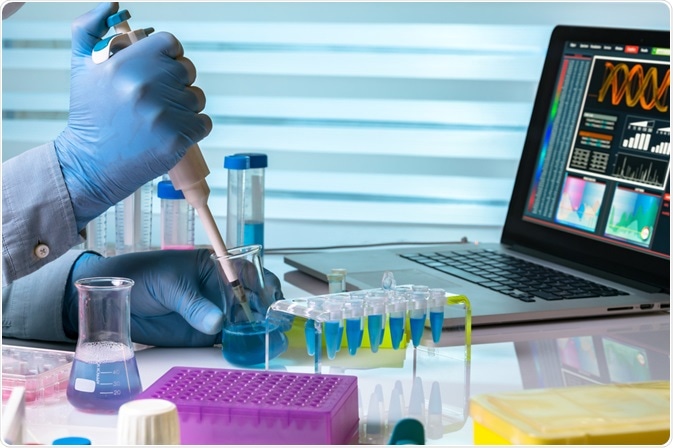
Image Credit: angellodeco/Shutterstock.com
Core Concepts and Branches of Analytical Chemistry
Analytical chemistry can be broadly divided into two types of analysis: qualitative and quantitative. Qualitative analysis involves identifying the specific components of a sample and determining what substances are present, without focusing on their quantities. Conversely, in quantitative analysis the concentration or amount of each component in a sample is determined.
Various techniques, or a combination of thereof, can be used, including spectroscopy, chromatography, and electrochemical analysis. These approaches allow the detection of substances at low concentrations (often down to parts-per-billion) making them crucial for applications requiring high sensitivity and specificity.
Regardless of the approach, a form of separation is generally required to isolate key components in the sample before they can be analyzed.
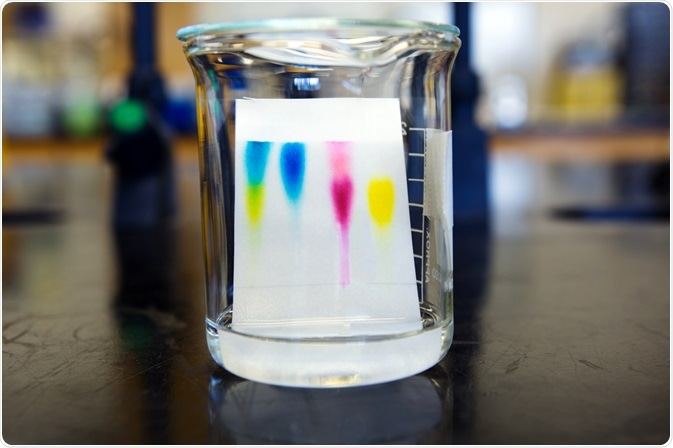
Image Credit: ggw/Shutterstock.com
Modern Techniques in Analytical Chemistry
In recent years, technological advancements have made analytical methods more accurate, efficient, and capable of handling complex samples. Among the most widely used techniques there are mass spectrometry (MS), nuclear magnetic resonance (NMR) spectroscopy, chromatography, and atomic spectroscopy.
MS measures the mass-to-charge ratio (m/z) of molecules. Instrumentation typically consists of an ion source where the sample is ionized, a mass analyzer where ions are sorted according to their m/z, and a detector, where ions are measured before being displayed as a mass spectrum. This technique is extensively used in pharmaceuticals, environmental testing, and proteomics.
NMR is a powerful tool for structural analysis, especially in organic chemistry, biochemistry, and materials science. Based on the magnetic properties of certain atomic nuclei (i.e., 1H, 13C, 15N, 31P) and how they behave when exposed to a magnetic field, NMR helps understand the molecular composition and structure of proteins, organic compounds, and polymers.
Chromatography allows the separation of compounds from a complex mixture based on the interactions the analytes have with a mobile phase (which can be a gas or a liquid) and a stationary phase (fixed on a column). As the constituents within the mixture have different retention times on the stationary phase, they are eluted at different rates, resulting in their separation.
There are several types of chromatography techniques, including liquid chromatography (LC), gas chromatography (GC), and high-performance liquid chromatography (HPLC). HPLC is widely used in pharmaceuticals for drug analysis, while GC is often employed for the detection of volatile pollutants in environmental testing.
Atomic spectroscopy, which includes techniques like atomic absorption and emission spectroscopy, is essential in materials science and environmental monitoring. These techniques can allow the detection of metal ions and trace elements, and are used in the determination of soil contamination or the composition of alloys.
What is Analytical Chemistry | Analytical Chemistry Methods | What does Analytical Chemists Do
Discover how AI is transforming the field
Applications of Analytical Chemistry
Analytical chemistry has broad applications across various sectors. Techniques like mass spectrometry and NMR are used to discover biomarkers, aiding in the diagnosis and monitoring of diseases. HPLC is applicable to complex samples such as plasma, urine, and other biological fluids.
The great resolution and reproducibility of HPLC allows for the separation, identification, and quantification of biomolecules such as proteins, lipids, hormones, and metabolites, and it is therefore a powerful tool for the discovery and monitoring of biomarkers.1
Analytical methods are crucial in environmental monitoring, where they help identify pollutants in air, water, and soil. Chromatography is widely used to analyze water quality and estimate potential health risks.2
Atomic absorption spectroscopy (AAS) and inductively coupled plasma (ICP) spectroscopy are used to determine the presence of heavy metals in soil. The analysis of CO2 and volatile organic compounds (VOCs) may be used to assess air quality.3
Ensuring the safety and authenticity of food products relies on analytical techniques to detect contaminants, verify quality, and prevent adulteration. Spectroscopy and chromatography, for example, are used to detect pesticides, food additives and nutritional components.4
In developing new materials, analytical chemistry is used to understand the composition and properties of substances like polymers, metals, and ceramics. Techniques like X-ray diffraction and electron microscopy provide detailed structural information crucial for innovation in materials research.
Find out more about analytical instruments for your lab
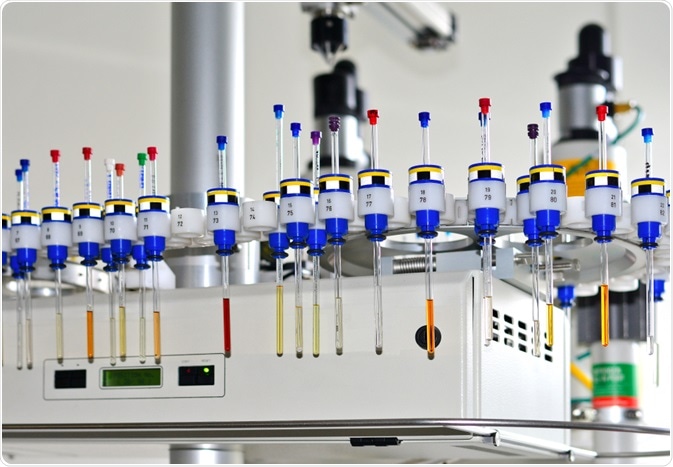
Image Credit: smereka/Shutterstock.com
Recent Advances and Trends
Technological advancements and recent innovations have contributed to the rapid evolution of analytical chemistry over the years. The development of handheld analytical devices has allowed for real-time, on-site testing in the field, particularly beneficial in environmental monitoring.5
Efforts have been made to automate methods and reduce analysis time to allow high-speed testing of numerous samples. Particularly, chromatography-based techniques and mass spectrometry are often used for high-throughput screening studies, essential for drug discovery and environmental analysis.6
Over the last few years, analytical chemistry has increasingly incorporated artificial intelligence and machine learning. These technologies offer unprecedented capabilities in interpreting large volumes of spectroscopic and chromatographic data.
There is also a lot of emphasis on addressing environmental problems with the development of green analytical chemistry, which aims to make analytical methods more sustainable. Eco-friendly practices are promoted by reducing the use of hazardous chemicals, energy, and waste, as well as through innovations in solvent-free techniques and alternative materials.
Challenges and Future Directions
As applications become more complex, there is an increasing need for more sensitive and precise analytical methods able to detect substances at very low levels – crucial for fields like toxicology and environmental science.
In many applications it is important to balance accuracy with speed and cost. Techniques must be optimized to provide rapid results without compromising accuracy. With ongoing innovations in instrumentation, automation, and AI integration, analytical chemistry will continue to evolve and will keep playing a central role in the advancement of other scientific fields too.
References
- Denoroy, L., Zimmer, L., Renaud, B. & Parrot, S. (2013). Ultra high performance liquid chromatography as a tool for the discovery and the analysis of biomarkers of diseases: A review. Journal of Chromatography B, 927, 37-53.https://doi.org/10.1016/j.jchromb.2012.12.005. Available: https://www.sciencedirect.com/science/article/pii/S1570023212007325
- Molnarova, L., Halesova, T., Vaclavikova, M. & Bosakova, Z. (2023). Monitoring Pharmaceuticals and Personal Care Products in Drinking Water Samples by the LC-MS/MS Method to Estimate Their Potential Health Risk. Molecules, 28.10.3390/molecules28155899.
- Szulc, J., Okrasa, M., Ryngajłło, M., Pielech-Przybylska, K. & Gutarowska, B. (2023). Markers of Chemical and Microbiological Contamination of the Air in the Sport Centers. Molecules, 28, 3560. Available: https://www.mdpi.com/1420-3049/28/8/3560
- Cortés-Herrera, C., Artavia, G., Leiva, A. & Granados-Chinchilla, F. (2018). Liquid Chromatography Analysis of Common Nutritional Components, in Feed and Food. Foods, 8.10.3390/foods8010001.
- Hutengs, C., Ludwig, B., Jung, A., Eisele, A. & Vohland, M. (2018). Comparison of Portable and Bench-Top Spectrometers for Mid-Infrared Diffuse Reflectance Measurements of Soils. Sensors (Basel), 18.10.3390/s18040993.
- Vervoort, N., Goossens, K., Baeten, M. & Chen, Q. (2021). Recent advances in analytical techniques for high throughput experimentation. Analytical Science Advances, 2, 109-127.https://doi.org/10.1002/ansa.202000155. Available: https://chemistry-europe.onlinelibrary.wiley.com/doi/abs/10.1002/ansa.202000155