By Pooja Toshniwal PahariaReviewed by Lexie CornerMay 6 2025
A recent study published in Science Advances introduces a new approach to three-dimensional (3D) printing that integrates cells and extracellular matrix (ECM) into biologically functional scaffolds.
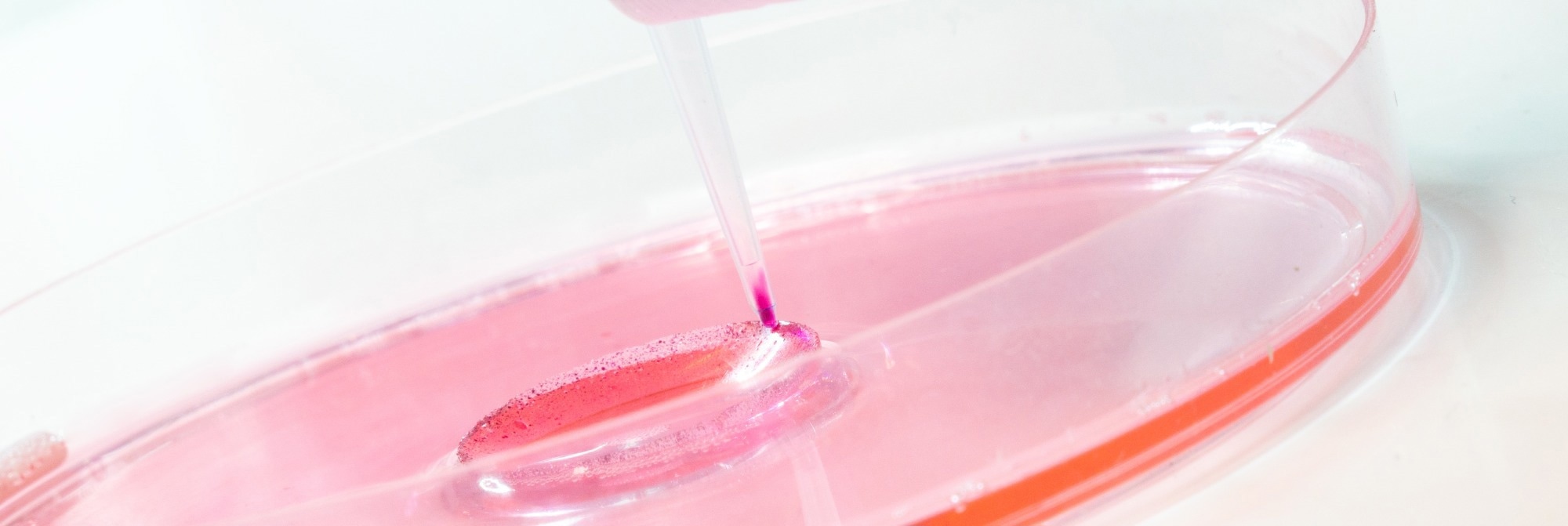
Image Credit: luchschenF/Shutterstock.com
These collagen-based, high-resolution, internally perfusable scaffolds, referred to as CHIPS, work in tandem with a customizable vascular and perfusion organ-on-a-chip reactor system (VAPOR). Together, they enable the formation of living tissues that support nutrient transport, cell migration, and viability.
The team created a pancreatic-like scaffold with embedded vascular channels capable of mimicking organ-level functions, offering a platform for disease modeling and potential cell replacement therapies.
Cardiovascular tissues are essential for transporting oxygen and nutrients throughout the body and clearing metabolic waste. Yet, bioengineering these systems remains challenging. One persistent issue is the difficulty of generating perfusable microvascular networks, which often limits tissue thickness and results in necrotic zones due to insufficient oxygen.
Microfluidic technologies have advanced efforts to model vascular systems and biological processes, providing a basis for studying angiogenesis, disease mechanisms, and drug responses. However, current fabrication methods often fall short when it comes to mechanical robustness, material compatibility, structural complexity, and supporting dynamic tissue remodeling.
About the Study
The research team developed a tissue engineering platform that combines CHIPS scaffolds with the VAPOR bioreactor, allowing for the growth of tissues that mimic native physiological function.
Using the Freeform Reversible Embedding of Suspended Hydrogels (FRESH) method, the team directly printed collagen scaffolds in a single step. The process involved extruding biomaterials into a thermo-reversible gelatin microparticle support bath, which stabilized the printed structures. By raising the temperature to 37°C, the gelatin melted, enabling the gentle recovery of intact printed scaffolds.
Print fidelity was assessed based on filament diameter and infill spacing, but the researchers also emphasized the importance of precisely distinguishing between positive spaces (occupied by cells and ECM) and negative spaces (channels for vascular or lymphatic perfusion).
To showcase the technology, they used multi-material 3D printing to fabricate pancreatic-like CHIPS seeded with murine insulinoma (MIN6) cells. These scaffolds were maintained in VAPOR bioreactors for 12 days to support nutrient delivery, cell proliferation, and insulin production in response to glucose fluctuations.
The multi-material setup allowed fine-tuned control over ECM composition, spatial arrangement, and cell placement. Using three ink extruders aligned via a dual-camera optical system, the team created centimeter-scale structures featuring complex microfluidic geometries, such as serpentine mixers, stacked channels, and tri-channel designs separated by narrow collagen walls.
Advanced imaging techniques, including optical coherence tomography (OCT), confirmed mechanical precision, while confocal and stereo microscopy visualized internal architecture. Real-time fluorescence tracked the diffusion of molecules ranging from 3 to 70 kDa, validating the perfusability of the scaffold networks.
Experiments with human umbilical vein endothelial cells (HUVECs) and bone marrow-derived mesenchymal stem cells (MSCs) confirmed robust cell adhesion and endothelial activity within the CHIPS framework.
Results
The integrated CHIPS-VAPOR approach comprises a highly modular tissue engineering platform validated by the pancreatic-like CHIPS forming capillary-like networks under perfusion culture. The method generated serpentine, triple, and stacked CHIPS using collagen type I with a root mean square (RMS) error of less than 20 μm. This level of detailing surpasses conventional bioprinting methods, enabling the design of intricate microfluidic networks.
A filament diameter of 105μm provided high mechanical strength and improved cell adhesion. Infill spacing with deviations of less than 10 μm indicated high repeatability. The measurements suggest that CHIPS can recapitulate the designed CAD model with high-fidelity positive features and perfusable channels. OCT imaging confirmed the patency of vascular networks with circular lumens and varying diameters. The findings highlight the ability of multi-material printing to design flexible CHIP systems.
The cell culture experiments showed that cells could preferentially migrate along printed collagen filaments within the infill of the CHIPS model. Molecules with different weights could diffuse through the CHIPS channels into the bulk of the scaffolds, a process difficult to achieve with current microfluidic systems comprising plastics or PDMS.
The pancreatic CHIPS produced more than 8.0 ng of insulin, and perfusion increased insulin expression. The team observed looping structures comprising insulin-secreting MIN6 cells and actin-secreting HUVECs and MSCs. These structures are reminiscent of early pancreatic morphogenesis occurring during pancreatic islet development.
Conclusions
This study presents CHIPS and the VAPOR system as a versatile platform for engineering biologically functional tissues at organ scale. The approach surpasses current extrusion-based bioprinting techniques in both resolution and fidelity, supporting detailed microfluidic designs and complex cell-ECM arrangements.
Key advancements include precise spatial control of scaffold architecture (±20 μm) and a direct printing method that eliminates the need for labor-intensive post-printing perfusion seeding. Future work may focus on structural reinforcements to improve long-term stability and integration with native vascular systems.
Download your PDF copy now!
Journal reference
Daniel J. Shiwarski et al. 3D bioprinting of collagen-based high-resolution internally perfusable scaffolds for engineering fully biologic tissue systems. Sci. Adv. 11, eadu5905 (2025), DOI:10.1126/sciadv.adu5905, https://www.science.org/doi/10.1126/sciadv.adu5905